REVIEW ARTICLE
Elisabetta Giovannini*, Maria Chiara Gaeta, Andrea Ciarmiello
Nuclear Medicine Unit, Sant’ Andrea Hospital, La Spezia, Italy
Abstract
Neuroendocrine tumors (NETs) include a spectrum of neoplasms characterized by histologic heterogeneity with significant clinical differences. Generally are well differentiated tumors but often present metastases at diagnosis. Conventional imaging techniques result insufficient in early diagnosis and therapy monitoring. Standardized morphological criteria to assess treatment response are inadequate in NETs, because of their biologic evolution and the cytostatic nature of new oncologic treatments. Functional imaging modalities have improved the understanding and diagnosis of NETs by the use of somatostatin analogue tracers labelled with radioisotopes. 111In-Octreotide scintigraphy was considered the gold standard imaging modalities for NET detection with a diagnostic accuracy approximately of 90%. Actually 68Ga-Dota-SST radiotracers (SSTRTs) PET/CT represent a superior imaging procedure with higher accuracy in detection of NET lesions as compared to morphological imaging procedures and somatostatin receptor scintigraphy. Additionally, the use of somatostatin analogue radiolabelled tracers offers the possibility to non-invasively evaluate the presence of somatostatin receptor expression on NET cells, with direct therapeutic implications. However, in the management of patients with NETs and in the evaluation of response to therapy the specialists opinions remain various. This review is based on a PubMed search of medical literature and presents the systems of classification, grading and staging of NETs. It also summarizes common recommendations for the management of patients with NETs, focused especially on the role of 68Ga-DOTA-SSTRTs PET/CT. In this review, the function of 68Ga-DOTA-SSTRTs PET/CT in pediatric neuroendocrine tumors is also explored.
Keywords: neuroendocrine tumors, 68Ga-DOTA-SSTRTs PET/CT, somatostatin receptor.
Introduction
Neuroendocrine neoplasms (NETs) are tumors with an incidence of approximately 5/100,000 per year. Annual incidence is increasing over the past decades due to improvements in diagnosis and the availability of a more sensitive biochemical evaluation. Therefore, although NETs are traditionally considered rare, their prevalence is higher than that for gastric, esophageal and pancreatic cancers. Despite the improved diagnostic workup, making a diagnosis of NETs is usually challenging and often delayed. As a result metastatic disease at diagnosis is common. The detection of NETs by conventional imaging, such as computed tomography (CT), ultrasonography (US), and magnetic resonance imaging (MRI) is limited [1]. Moreover, even in those patients with localized disease, the prognosis is not favourable with a five-year survival rate of less than 80% because of a high risk of recurrence in a not negligible proportion of patients [2–3].
Biology
Neuroendocrine neoplasms originate from neuroectoderma or endoderma cells and have both neural and endocrine cell features. It is well known that NETs can develop in almost all tissues or organs in the body, especially in the gastroenteropancreatic tract and lungs and rarely in the ovary [2]. They are characterized by an ample range of histological appearance and biological behavior These tumors show tissue immunoreactivity for markers of neuroendocrine differentiation (synaptophysin, chromogranin A, CD56, and NSE) and may secrete various peptides and hormones [4]. NETs are frequently placed in the submucosa or more deeply intramurally and can invade surrounding tissues. The tumoral cells can be organized variously in islands, glands or sheets.
Often neuroendocrine cells show minimal pleomorphism but they can evolve in anaplasia andrapid mitotic activity leading to intratumoral areas of necrosis. Some neuroendocrine tumor cells express hormone receptors with a strong avidity for specific hormones that can be exploited for diagnosis and treatment purposes. In particular, neuroendocrine tumors possess somatostatin receptors (SSTRs) with a very variable expression in terms of density and subtypes. Five subtypes of SSTRs, SSTRs 1-5; have been cloned and they belong to a distinct group within the superfamily of G-protein-coupled receptors with seven transmembrane regions [5].
A high density of somatostatin receptors is found in neuroendocrine tumors, such as pituitary adenoma, gastroentropancreatic tumors, carcinoid, pheochromocytoma, paraganglioma, medullary thyroid cancer, and small cell lung carcinoma. Tumors of the nervous system including meningioma, neuroblastoma, and medulloblastoma also often express a high density of SSTR. Tumors not originating from the neural or endocrine cells, such as lymphoma, breast cancer, renal cell cancer, hepatocellular carcinoma, prostate cancer, sarcoma and gastric cancer, may also express SSTR. The expression of SSTR is not specific for malignancy. Some benign lesions may also express SSTR, for example, active granulomas in sarcoidosis [6].
Classification of neuroendocrine tumors
A consensus about a recognized uniform grading system for neuroendocrine neoplasms has been difficult to achieve. The systems proffered by the American Joint Committee on Cancer (AJCC), World Health Organization (WHO), European Neuroendocrine Tumor Society (ENETS) and others provide useful prognostic information [1,7].
Neuroendocrine neoplasms can be classified by: anatomic site of origin; histology: well-differentiated (G1 and G2) NETs, poorly differentiated (G3) carcinomas or undifferentiated neoplasms; extent of disease: local, regional or distant metastases.
Most NETs arise from the GI tract (stomach, appendix, duodenum, and small intestine), the bronchopulmonary system (lungs and thymus), the pancreas, the colon and rectum. The biological behaviour exhibited by neuroendocrine neoplasms is highly correlated with neoplasm grade. The grade of a tumor refers to its biologic aggressiveness. The grading system is based on the rate of proliferation, which is defined by the number of mitoses per 10 high-power microscopic fields or per 2 mm2 (mitotic rate), or as the percentage of tumor cells that immunolabel positively for the Ki-67 antigen (Ki-67 index). Briefly, low-grade tumors are characterized by low proliferative indices and are considered indolent in nature. High-grade tumors tend to be poorly differentiated, have high proliferative indices and are therefore very aggressive [8] (Table 1).
Table 1. Grading Systems for Neuroendocrine.
For neuroendocrine neoplasms, the presence of necrosis also plays an important role in grading. For example, G1/G2 bronchial NETs (typical/atypical carcinoids) and G3 bronchial neoplasms (large cell neuroendocrine carcinoma [LCNEC] and small cell lung carcinoma [SCLC]) exhibit markedly different behavior. The presence of necrosis, alongside mitotic activity, is a distinguishing feature between these 2 groups of tumors. NETs can also be classified based on differentiation, referring to the extent to which neoplastic cells resemble normal cells.
In general, the WHO guidelines divide neuroendocrine neoplasms into 2 clinically distinct pathologic classes: well– and poorly differentiated. Well-differentiated NETs can be classified as either grade 1 or grade 2 depending on proliferation and histology. Well-differentiated grade 1 and grade 2 NETs traditionally have been referred to as carcinoids, regardless of grade or site of origin. Poorly differentiated grade 3 neuroendocrine carcinomas are characterized by rapid dissemination, resistance to therapeutic interventions and a highly aggressive course.
Table 2. Nomenclature for Neuroendocrine Neoplasms.
Well differentiated NETs have a typical organoid arrangement of cells with nesting, trabecular, or gyriform patterns. Well differentiated NET cells produce large amounts of secretory granules with diffuse immunoexpression of neuroendocrine markers. In contrast, poorly differentiated NETS have atypical, sheet-like, diffuse and irregular nuclei, less cytoplasmic secretory granules and limited biomarker immunoexpression. Well differentiated NETs are usually of low or intermediate grade whereas poorly differentiated NETs are usually high grade. The histological classification of NETs, including grade (G) and differentiation is outlined in Table 2.
According to the WHO classification 2004, NETs of the lungs share common morphological, immunohistochemical and molecular characteristics. They can be divided into three main entities (Table 3): Carcinoid tumors (typical (TC)/atypical (AC)); large cell neuroendocrine carcinomas (LCNEC); small cell carcinomas (SCLC).
Table 3. Macroscopical, histological and cytological features of pulmonary NETs.
These neuroendocrine entities are further classified into two groups; according to their biological aggressiveness [9]: well-differentiated low grade (G1) typical and intermediate grade (G2); atypical carcinoids; poorly-differentiated high grade (G3) LCNEC and SCLC. In contrast with typical and atypical carcinoids, LCNEC and SCLC are not closely related to each other regarding the genetic and epigenetic characteristics. Unlike carcinoids, no precursor lesions are known for SCLCs and LCNECs [10–12].
In typical carcinoids, regional lymph node metastases can be found in 10–15% and distant metastases in 3–5% [13]. In atypical carcinoids, nodal metastases are found in 50% and distant metastases in 25%. As metastases of G1 and G2 NETs are not sensitive to chemotherapy, surgery remains the first choice of treatment in metastatic diseases. Due to the high metastatic risk (in 50% to 80%) of these tumors, prophylactic cranial radiation is usually done [14].
Small cell lung cancers because of differences in clinical behavior, therapy and epidemiology are classified separately. Several staging systems have been proposed for small cell lung cancer (SCLC) by American Joint Committee on Cancer (AJCC), WHO, Veterans Administration Lung Study Group (VALG) and International Association for the Study of Lung Cancer (IASLC) [15-16]. The staging systems include two levels according to metastasis: Limited-Stage Disease (LD) (disease is confined to the hemithorax of origin, the mediastinum or the supraclavicular nodes, which can be encompassed within a tolerable radiation therapy port).
Patients with pleural effusion, massive pulmonary tumor, and contralateral supraclavicular nodes have been both included within and excluded from LD by various groups. Extensive-Stage Disease (ED) (SCLC has spread beyond the supraclavicular areas and is too widespread to be included within the definition of LD. Patients with distant metastases (M1) are always considered to have ED) [15–16]. This distinction is crucial because the shape of limited disease responds very well to chemotherapy associated with radiotherapy, especially with drug combinations that use the cis-platinum. In general surgery is not recommended [17].
More recently the WHO classification of the neuroendocrine neoplasms of gastroentropancreatic (GEP) system subdivided them into two major categories: neuroendocrine tumor (NET) and neuroendocrine carcinoma (NEC), based on histological differentiation (well differentiated and poorly differentiated), proliferative activity (G1, G2 and G3) and TNM factors (size, infiltration/invasion and metastasis). NET G1 and NET G2 correspond to what were formerly called well differentiated endocrine tumor and well differentiated endocrine carcinoma, respectively. NEC G3 is nearly the same as poorly differentiated endocrine carcinoma and highly malignant [18–19] Table 2.
The European Neuroendocrine Tumor Society and the American Joint Committee on Cancer stage NETs by the primary tumor (T), lymph node involvement (N), and distant metastasis (M). This TNM staging represents a new system of NET classification. The definition of TNM varies by each primary tumor site; however, staging relies predominantly on the size of the tumor and the extent of invasion into anatomical structures. For example, a NET in the colon invading the muscularis propria with no lymph node involvement or distant metastases would be considered Stage IIA or IIB.
The North American Neuroendocrine Tumor Society (NANETS) recommend that pathology reports provide a TNM stage, based on a system that is specifically referenced in the pathology report [19–20]. In the NET G1, G2 group, SSTR-2A expression is significantly higher in the gastrointestinal primaries than in the lung primaries. This may suggest that SSTR-2A is a receptor subtype, characterizing the low grade neuroendocrine neoplasms of GI origin. Among the SSTR subtypes, the expression of SSTR-2A was significantly high in both the NET and NEC groups. No significant difference was observed in the expression pattern of SSTR subtypes in the NET and NEC groups.
However, a significant difference in the expression profiles of SSTR-1 and 2A between NEC G3 small cell type and non-small cell type was obtained. In NEC G3 small cell type, the expression of SSTR-1 and 2A was significantly low. Alternatively, the expression of SSTR-5 is rather high leading to the conclusion that NEC G3 should be classified into small cell type and non-small cell type for example lung cancer.
All the specific antibodies against SSTR-1, 2A, 3 and 5 show specific and satisfactory immunolocalization of SSTR subtypes in the tumor cells. The immunolocalization of SSTR-2A is usually membranous and intensely stained. Regarding the expression of SSTR-2A in the NET G1, G2 group, there is relatively good correlation between the expressions of mRNA (100%) and protein (>80%). However, in the NEC G3 group the expression of SSTR-2A protein is rather low (61.9%) compared with the expression of mRNA (95.2%). Such discrepant results may be caused by the different sensitivities of each detection system.
The immunohistochemical localization of SSTR-1, 3 and 5 is usually cytoplasmic, but membranous localization is also seen occasionally. No significant correlation is observed between the expression of neuroendocrine markers (synaptophysin, chromogranin A, CD56, and NSE) and the expression patterns of SSTR subtypes [21]. The expression of SSTR represents the bases of molecular imaging.
Imaging modalities
Morphological or structural imaging technologies available for assessment of NETs include CT, MRI, and ultrasonography. These imaging techniques are used especially to assess the morphologic features, to identify changes in the target lesions over time and to detect new tumors through use of serial imaging. Each of these modalities has advantages and disadvantages dependent by tumor characteristic and location.
Computed tomography
Computed tomography (CT) is the modality of choice for the initial imaging work-up and for therapy monitoring of NET disease. Radiologically, pulmonary carcinoids (TC ≤ 2 cm and AC ≥ 4 cm) are nodules or masses (TC with a smooth margin and AC with an irregular margin). Approximately 30-55% of carcinoids cause lobar atelectasis, obstructive pneumonitis and partial obstruction. Their visualization can improve with intravenous administration of contrast due to the marked enhancement of their vascular stroma [22].
In SCLC usually a central mass formed by the combination of primary tumor and lymph node metastases can be found radiologically. Mediastinal lymph node involvement is present in most cases (5% to 10% present as a peripheral nodule without lymph node involvement). Reduction and displacement of major vessels and bronchi and pleural effusion are common findings.
Contrast-enhancement CT examination is especially important in early disease for pre-operative planning. Three-dimensional (3D) volume rendering technique (VRT) and maximum intensity projections (MIPs) in CT angiography is useful preoperatively to visualize arterial anatomy and detect vascular encasement.
The high spatial resolution of current CT scanners allows to show the relation between the pancreatic duct and tumor in the GEP [23–24]. In general for abdominal imaging, MRI is superior to CT because of the better soft tissue contrast. On the other hand, the better spatial resolution of CT is more advantageous for detection of small lung metastases and therefore for examination of the thorax CT rather than MRI should be performed [25].
Magnetic resonance imaging
For MRI, specific imaging protocols for NETs have been described, including hyperintense signal intensity on fluid-fluid levels on T2-weighted (T2w) imaging and arterial hypervascularity. Intra vascular contrast enhancement is mandatory in MRI for NETs imaging and it is recommended that the liver and pancreas should be always examined by so called ‘triple-phase scanning’. The examination is performed before and during i.v. contrast enhancement in the late arterial phase (portal-venous inflow phase) and venous (portal-venous) phase. Magnetic resonance cholangiopancreatography (MRCP) is too generally included in NET evaluation, allowing better visualization of the main bile duct and the pancreatic duct also respect to CT [26].
Recent studies suggest diffusion–weighted (DW) MR sequences to be very sensitive for the detection of NET and for characterization of metastases, particularly helping in identify small lesions [27–28]. Additionally, functional MRI examinations (dynamic enhancement contrast MRI: DCE-MRI) with the introduction of a particular contrast enhancement, gadolinium-EOB-DTPA, has increased sensitivity and specificity, particularly for the detection of liver metastases. DCE-MRI is able to quantify the microcirculatory status of the liver prospecting the possibility to go beyond the mere morphologic assessment [28].
Somatostatin receptor imaging
Over-expression of somatostatin receptors (SSTR) is the rationale for molecular imaging in NET. The receptors are expressed in about 80–90% of NETs [20], particularly in well-differentiated neuroendocrine tumors. All the subtypes of SSTR expressed by NET have affinity for the native peptide. Hence, the sensitivity of somatostatin receptor studies depends on the density of the SSTR in the tumor and the type of analogue used. Somatostatin receptors are expressed by many neuroendocrine and non neuroendocrine cells of the body, so different organs may be imaged by somatostatin receptor scintigraphy including the liver, spleen, pituitary, thyroid, kidneys, adrenal glands, salivary glands, stomach wall, bowel [29–30].
Scintigraphy
Scintigraphy (SRS) using 111In-DTPA-octreotide (Octreoscan) is the standard method. Whole-body images (anterior and posterior views) are acquired at 4 and 24 h and single photon computed emission tomography (SPECT or SPECT/CT) of the abdomen and thorax is performed 24 h after injection. The sensitivity and specificity of SRS is varying in different reports, although a review comprising 35 centers and 1200 patients showed median 89% (range 67 — 100%) detection rate and median 84% (range 57 — 93%) sensitivity, but the sensitivity at SRS varies with the tumor type and its anatomical localization [31–32].
PET/CT-68Ga-DOTA-SSTRTs
The introduction of 68Ga-DOTA-SSTRTs PET/CT for the evaluation of NETs has significantly improved the diagnostic work-up, previously based only on conventional imaging (CI) modalities (ultrasound, CT, endoscopy, MRI) and somatostatin receptor scintigraphy (SRS) [33–34]. 68Ga-DOTA-conjugate peptides are rapidly cleared from the blood. Maximal tumour activity accumulation is reached 70+/-20 min post injection. Excretion is almost entirely through the kidneys [35]. Exist various 68Ga-peptide preparations (68Ga-DOTATOC, 68Ga -DOTANOC, 68Ga DOTATATE) which show different affinity to the somatostatin receptor subtypes but appear to be similarly effective in visualizing NETs in patients [6]. 68Ga-DOTATOC binds to SSTR5 with intermediate affinity and 68Ga-DOTANOC has high affinity to SSTR2, SSTR3, and SSTR5 [36].
Functional imaging by PET/CT with 68Ga-labeled octreotide and octreotate (68Ga-DOTATOC, 68Ga-DOTATATE, 68Ga-DOTANOC), has shown excellent results in NET patients. In a large number of studies, PET and PET/CT with these tracers have shown superior to morphologic imaging, mostly in comparison with CT. A large prospective study also demonstrates a higher accuracy of 68Ga-DOTATOC in comparison to the anatomical imaging modality, CT, and conventional SRS [37]. The advantages of PET over SRS are a higher tissue contrast and a better spatial resolution of about 0.5 cm compared to 1–1.5 cm with SPECT and planar scintigraphy.
Furthermore, with the 68Ga-preparations there are logistical advantages because of their more favorable kinetics that allows PET imaging already 1 h after injection [2,38]. The first clinical investigation on SSTR targeting using 68Ga-DOTATOC PET was published in 2001 by Henze et al. [39], who studied patients with meningiomas. The hypothesis of the study was that PET imaging with 68Ga-DOTATOC could help differentiating between meningiomas, neurofibromatosis and metastases, since meningiomas highly express SSTR2. They imaged three patients with 68Ga-DOTATOC PET, who had a total of eight meningiomas between them. Dynamic PET images of the demonstrated rapid radiopharmaceutical uptake within these tumors.
Quantitative analysis showed that the standard uptake value (SUV) increased immediately after injection, and reached a plateau 60–120 min after the injection (mean SUV¼10.6). There was no radiopharmaceutical uptake in adjacent healthy parenchyma, and even the smallest lesions (7–8 mm) showed high uptake with very high tumor to background ratios. This study provided useful information about the extent of tumor spread relative to adjacent osseous structures, especially at the base of the skull. Henze et al. [40] studied meningiomas with dynamic 68Ga-DOTATOC PET to evaluate the utility of obtaining radiotracer kinetic parameters prior to radiotherapy. They performed dynamic PET studies in 21 patients with a total of 28 lesions.
They demonstrated significant differences between meningiomas and the reference tissue (nasal mucosa) in the mean SUV (10.5 vs 1.3), and in the kinetic parameters such as vascular fraction (vB), rate constants k2, k3, k4 (1/min) and receptor binding (k1–k1/k2). These factors resulted in very high tumor-to-background ratios, allowing clear visualization of lesions at the skull base, particularly important for radiotherapy planning.
There have been several studies using 68Ga-DOTATOC PET for the detection of SSTR- positive malignancies, including metastatic lesions [37]. Hofmann et al. compared 111In-octreotide scintigraphy with 68Ga-DOTATOC PET in eight patients with histologically proved carcinoid tumors. They studied a total of 40 lesions that were identified either by CT and/or MRI. In total 68Ga-DOTATOCPET identified 100% of these lesions, whereas 111In-octreotide planar and SPECT imaging identified only 85% of the lesions.
Quantitative analysis showed that 68Ga-DOTATOC PET imaging resulted in higher tumor-to-non-tumor contrast with lower renal accumulation compared to 111In-octreotide. Kowalsk et al. [41] similarly presented a comparison between 68Ga-DOTATOC PET and 111In-DTPA-octreotide imaging in four patients with NET. 68Ga-DOTATOC PET appeared superior especially in detecting small tumors or tumors bearing only a low density of SSTRs. Both 111In-DTPA-octreotide SPECT and 68Ga-DOTATOC PET were less sensitive in the detection of liver metastases of NET compared to CT because they demonstrated lower overall uptake than the surrounding liver.
NETs are rare lesions that occur most commonly in the gastrointestinal tract and express a mine and peptide receptors, e.g. SSTR and receptors for vaso intestinal peptide, bombesin, cholecystokinin, gastrin, and/or substance P.). A recent study addressing the use of 68Ga-DOTATOC PET in NET was reported by Gabriel et al. [33]. They compared 68Ga-DOTATOC PET with 99mTc-HYNIC-octreotide and 111In- DOTATOC scintigraphy and CT in 88 patients with known or suspected NET. Patients were placed into one of three categories: those with an unknown primary tumor, but with clinical or biochemical suspicion of neuroendocrine malignancy (13 patients); those for staging of known tumor (36 patients); and those being followed up after therapy (35 patients). 68Ga-DOTATOC PET demonstrated significantly better diagnostic efficacy with a sensitivity of 97%, a specificity of 92% and accuracy of 96%.
Furthermore, the combined use of PET and CT gave the highest overall accuracy. Buchmann et al. [42] compared the relative utility of 68Ga-DOTATOC PET and 111In-DTPA-octreotide SPECT in the detection of NET and its manifestations. In that study (25 patients), SUVs of positive lesions on 68Ga-DOTATOC PET were from 0.7 to 29.3 for the mean SUV and from 0.9 to 34.4 for maximum SUV, while tumor/normal tissue ratios of 111In-DTPA-octreotide SPECT ranged from 1.8 to 7.3. In imaging of lung and skeletal lesions, 68Ga-DOTATOC PET was superior to 111In-DTPA-octreotide SPECT while in regional comparison of liver and , was identical.
The authors concluded that 68Ga-DOTATOC PET is superior to 111In-DTPA-octreotide SPECT in the detection of NET in the lung and skeleton and similar for the detection of NET in the liver and . Compared to 68Ga-DOTATOC, which is more specific for SSTR2, 68Ga-DOTANOC possesses a certain degree of selectivity for SSTR2, SSTR3 and SSSTR5 and demonstrates more favorable dosimetry [43]. Similarly, the radiotracer 68Ga-DOTANOC shows a high sensitivity in the detection of small lesions, particularly in liver, within lymph nodes and within bone metastases [44–46].
PET/CT-18F-FDG
Change in biology of the tumors is a known phenomenon and is attributed to either a change in the tumor receptor density or expression of a new receptor. Delineating these receptor changes may help defining patient’s prognosis and management [34]. Patients on follow-up with clinical or biochemical suspicion of a recurrence evaluated with 111In-DTPA-octreotide SPECT with poor to absent SSTR expression raise the probability of altered receptor status. NET is a well-differentiated pathology and does not express glucose transporter (GLUT) receptors and hence a 18F-FDG PET/CT study is not utilized in the work up. Dedifferentiating tumors show an increase in the GLUT receptor expression with a decline in the somatostatin receptor density; hence, an 18F-FDG PET/CT study would be efficacious in locating sites of tumor spread [47].
Combining receptor imaging studies with 18F-FDG PET/CT study may help in staging the disease as per the WHO classification which is based on the histology–type 1a: Well-differentiated benign, type 1b: Well differentiated with low-grade malignancy, and type 2 poorly differentiated. The prognosis of the tumor is dependent on the differentiation of the tumor, poorly differentiated having a bad prognosis [46]. In many patients there were a large number of tumor lesions, often with multiple lesions in the same organ (e.g., liver metastases). In some patients there was variable uptake of tracer at different lesion sites. Moreover, heterogeneous uptake within tumor lesions indicates that percutaneous biopsy may not fully reflect in vivo tumor heterogeneity. Despite these limitations, which to a large extent are inevitable in an imaging study, tumor grade and proliferation appeared to be related to tumor 68Ga-DOTATATE and 18F-FDG uptake.
68Ga-DOTANOC PET/CT was superior to 18F-FDG PET/CT in patient based analysis. In region based analysis, 68Ga-DOTANOC PET/CT results superior to 18F-FDG PET/CT in detecting lymph node metastases; no statistically significant differences there are in liver and skeletal lesions detection. Their role is complementary and the combination of 68Ga-DOTA-NOC PET/CT and 18F-FDG PET/CT helps defining the total disease burden and selecting patients for a proper therapeutic groups [48].
Clinical indication to perform 68Ga-DOTA-conjugate peptides PET/CT
In the management of NETs 68Ga-DOTA-conjugate peptides PET/CT is used to: localize primary tumours and detect sites of metastatic disease (staging) [49]; follow-up of patients with known disease, if at the first diagnosis the tumor showed SST receptor, to detect residual, recurrent or progressive disease (restaging) [31,42–43]; determine SST receptor status (patients with SST receptor-positive tumors are more likely to respond to Octreotide therapy) [46]; select patients with metastatic disease for SST receptor radionuclide therapy (with 177Lu or 90Y-DOTA-peptides ) [46]; monitor the response to therapy (surgery, radiotherapy chemotherapy or SST receptor radionuclide therapy) [7].
Staging
Numerous studies assessed the role of 68GA-DOTA in NET. Ambrosini et al. [47,50] in a study included 90 patients with pathologic confirmation of NET, exploring the clinical impact of 68Ga-DOTANOC PET/CT compared with CT for staging and change of therapy. Considering all cases, 68Ga-DOTANOC PET/CT changed both stage and therapy in 50 of 90 (55.5%) patients. The most frequent impact on the management (27 patients) was the initiation or continuance of peptide receptor radionuclide therapy, followed by the initiation or continuance of somatostatin analog medical treatment (7 patients) and referral to surgery (6 patients). PET prevented unnecessary surgery in 6 patients and excluded from treatment with somatostatin analogues 2 patients with NET lesions that did not express somatostatin receptors.
Less frequent impacts on management included the initiation of radiotherapy (1 patient), further diagnostic investigation (1 patient), and liver transplantation (1 patient). The Authors concluded highlighting the importance of the clinical role of PET in the management of NET [47].
Rakesh et al. [48] evaluated the role of 68Ga-DOTATOC PET/CT for detection and staging of pancreatic NETs. They enrolled twenty patients with clinically suspected and/or proven pancreatic NET. Patients underwent 68Ga-DOTATOC PET/CT, CT with contrast enhancement (ceCT), and 18F-FDG PET/CT for staging and/or localizing the primary lesion.
The imaging results were compared with histopathology and with clinical follow up. 68Ga-DOTATOC PET/CT correctly localized primary tumor in all 20, ceCT in 15 and 18F-FDG PET/CT in 2 patients. Additionally 68Ga-DOTATOC PET/CT detected metastases in 13 patients, ceCT in 7 and 18F-FDG PET/CT in 2. They concluded that 68Ga-DOTATOC PET/CT is a very useful imaging investigation for diagnosing and staging pancreatic NET.
Haug et al. tested the 68Ga-DOTATATE PET/CT as a diagnostic tool to improve the detection of tumor in a cohort of patients with suspected, no localized NET. 68Ga-DOTATATE PET/CT was performed in 104 consecutive patients with clinical suspicion of NET. Presence of NET was histologically verified in 36 cases. 68Ga-DOTATATE PET/CT identified NET in 29 of the 36 cases and excluded the presence of a NET in 61 of the 68 non-NET patients, indicating a sensitivity of 81% and specificity of 90%. PET/CT gave a false-positive result in 7 patients and a false-negative in another 7 patients, indicating positive and negative predictive values of 81% and 90%, respectively, and an accuracy of 87%.
The conclusion was that 68Ga-DOTATATE PET/CT is highly accurate in patients with clinical symptoms of NETs or elevated levels of tumor markers and should be used in clinical routine diagnostics [49].
Sharm et al. investigate about the predictive value of 68Ga-DOTANOC PET/CT in patients with suspected NET. They studied 164 patients who underwent 68Ga-DOTANOC PET/CT. Histopathology (n = 55) and clinical/imaging follow-up (n = 109; median, 11 months) was used as reference standard. Primary tumor was demonstrated in 90 patients (commonest site-pancreas) and metastasis in 30 (commonest site-liver). PET/CT was true positive in 92 patients, true negative in 58, false positive in 9, and false negative in 5. The overall sensitivity was 94.8%, specificity was 86.5%, positive predictive value was 91%, negative predictive value was 92%, and accuracy was 91.4%.
The Authors conclude that 68Ga-DOTANOC PET/CT shows high positive and negative predictive values in patients with suspected NET and can be routinely used for this purpose [51]. Naswa et al. considering that many small lesions and metastases of NET remain occult on CT evaluated the diagnostic performance of 68Ga-DOTANOC PET/CT in gastrinoma patients with negative or equivocal CT findings (n: 25). Diagnostic performance of 68Ga-DOTANOC PET/CT was superior in patients with equivocal ceCT findings than that in patients with negative ceCT. 68Ga-DOTANOC PET/CT showed more accuracy than ceCT [52].
Therapy
Although the neuroendocrine tumors tend to have a slow growth, often they are diagnosed in the metastatic phase. The wide range of type of neuroendocrine tumors implies the need for a variety of treatments. Therapy is typically multidisciplinary and must be adapted to each patient according to the tumor type, the extent of disease and symptoms. Surgery remains the primary modality for cure in patients with limited disease, early-stage disease. When this is not possible, medical therapy with somatostatin analogs are the mainstay for control of hormone-related symptoms associated with NETs as well as suppression of disease progression.
In addition to symptom control, treatment with long-acting somatostatin analogues has demonstrated antitumor activity, prolonging time to disease progression in patients with metastatic NETs. The targeted therapies, including the oral mTOR inhibitor everolimus and the tyrosine kinases inhibitor sunitinib have been shown to improve progression-free survival in patients with progressive malignant disease. Peptide receptor radionuclide therapy (PRRT) has also shown promising results in patients with disseminated disease. A high prevalence of somatostatin receptor expression among NETs provides the rationale for peptide receptor–targeted therapy as a treatment modality in patients with inoperable or metastatic disease. The most frequently used radionuclides for targeted radiotherapy in NETs are indium (111In), yttrium (90Y), and lutetium (177Lu), which differs in emitted particles, energy and tissue penetration [52–53]. Both the yttrium– and the lutetium–labeled compounds have demonstrated promising results in patients with NET [54–55].
Treatment response
Imaging plays a pivotal role in follow-up surveillance and assessment of response in patients with NETs. However, the complex clinical course of NETs and cytostatic nature of many NET treatments pose specific challenges for the assessment of response. The pitfalls of conventional morphologic imaging in evaluating disease after treatment are well established. Inherent difficulties include identifying and monitoring small volume disease, defining and reproducibly measuring individual large volume of lesions and time lag before tumor decrease occurs after the start of chemotherapy and radiotherapy.
Additionally, a discrepancy between patient outcomes and decrease in tumor size has been well documented in patients with gastrointestinal stromal tumors treated with imatinib. Similarly, the limitations of RECIST in predicting survival have been noted in patients with advanced hepatocellular carcinoma treated with sorafenib. In the SHARP trial, only 2% of patients in the treated group demonstrated a partial response by RECIST despite improvement in overall survival. These findings suggest that tumor shrinkage may not predict poor outcome in patients treated with targeted therapies because tumors may respond to targeted therapy by undergoing necrosis or cystic changes without decreasing, and possibly even increasing, in size.
Residual masses or fibroses that do not contain viable tumor can cause further uncertainty [20,29]. Functional imaging providing information on tumor physiology may have a great potential for measuring treatment response in patients in whom tumor variation of volume is not predictable. Response to targeted therapies that demonstrate cytostatic versus cytocidal effects may be associated mainly with a decrease in metabolism, even in the absence of a major reduction in tumor size.
Therefore, changes in functioning or metabolic characteristics of the tissue may be more predictive of outcome than the tumor size criteria used by RECIST. Physiologic changes can be detected much earlier than changes in size, allowing to evaluate previously response to therapy. Several functional imaging techniques including dynamic contrast-enhanced (DCE) MRI, diffusion weighted MRI (DW-MRI), PET/CT and SPECT/CT have demonstrated promising ability to provide quantitative information regarding the change of molecular characteristics of tumors after treatment [22,29]. Somatostatin receptor imaging is used for monitoring NET treatment and is valuable as a complement in patients with biochemical or clinical progressive disease for the detection of new lesions [53]. However, there are no unique opinions about the role of somatostatin receptor PET/CT imaging in treatment monitoring, particularly for therapy with analogue of somatostatin.
Similarly there are no specific references in the guidelines of NET probably because studies with a large number of patients remain to be performed [54]. Based on the possible limitations of SRS and 68Ga-DOTATOC PET/CT for monitoring therapy with analogues of somatostatin, in patients with well–differentiated NETs, other tracers could be considered for imaging such as 18F-dopamine, 125I-iodine-alphamethyltyrosine and 11C-5-hydroxy-tryptophan (11C-5-HTP) [58].
In a recent study of 61 patients, 18F-DOPA-PET/CT showed 91% sensitivity and 96% specificity for NET detection and resulted in a change of therapy in more than one quarter of the patients [55]. In a larger study of 82 patients, the accuracy of 18F-DOPA-PET/CT for initial NET localization and staging, follow-up and diagnosis of recurrent disease was confirmed [56].
In comparative studies, however, the use of 68Ga-labelled octreotide rather than F-DOPA has been shown to be more advantageous for NET visualization [60]. Although published studies measuring labeled L-DOPA or 5-HTP uptake before and during treatment for monitoring therapy in NET are lacking, interesting results have been noted from small studies in the setting of therapeutic monitoring. Currently, the feasibility of 5-HTP-PET and L-DOPA-PET for therapeutic monitoring in NETs is limited by the few centers that have the capability to perform the procedure [58].
Recurrence
68Ga-DOTATATE PET/CT is accurate in detection of recurrent NET after surgery. When it is possible, complete surgical resection with curative intent of the primary tumor should be performed; however, many patients may develop disease recurrence. The probability of recurrence may vary depending on the site and the biologic aggressiveness of the tumor. In literature definitive studies evaluating recurrence rates and median time to tumor recurrence after resection and eventually adjuvant regimens are scarce.
However data regarding recurrence after surgery should help identifying patient subgroups at particularly high risk of recurrence. Nevertheless, in patients with primarily localized stages, there is potential for improving 5-year survival rates, which remain less than 80%, because of recurrence in a comparably high proportion of patients. In a cohort of patients with pancreatic NET, 42% developed recurrent disease, manifesting in most cases with hepatic metastases after curative resection of the primary tumor, while in a cohort of patients with small-bowel NET, 59% developed recurrent disease, with a median time to recurrence of 32 months. Despite the considerable risk of development of recurrent disease in patients with NET, there is no consensus about the most favorable imaging modality for postsurgical follow-up, best intervals and duration of follow-up.
Positron emission tomography (PET) findings with 68Ga-labeled somatostatin analogs enables a good correlation with expression of somatostatin receptor type 2, which is typically high in resected NETs [61]. PET with somatostatin analogs is superior to conventional imaging and 111In-pentetreotide SRS, with a positive effect on patient treatment and prognostic accuracy. Recently, one study showed the high value of 68Ga-tetraazacyclododecane tetraacetic acid–octreotate (68Ga-DOTATATE) PET/CT in patients who are suspected of having a NET, such that it is now recommended by the guidelines of the European Neuroendocrine Tumor Society [57]. Some of these studies have also included patients during follow-up after curative surgery and 68Ga-DOTATATE PET/CT proved a high accuracy in detection of recurrent NET after surgery however lack of specific and consistent data.
Pediatric NET
Neuroendocrine tumors arising from the neural crest, such as neuroblastomas, form a large proportion of childhood malignancies, accounting for 7% to 10% of all pediatric neoplasms, while gastroenteropancreatic-NETs form a small subgroup of neural crest tumors whose imaging findings are not well-described in children. Certain tumors occur as part of hereditary syndromes such as multiple endocrine neoplasia types 1 and 2, von Hippel-Lindau disease, neurofibromatosis type 1, Carney complex, pheochromocytoma-paraganglioma syndrome and familial medullary thyroid carcinoma.
These syndromes generally appear at a young age and are characterized by specific genetic abnormalities. The combined use of molecular and anatomical imaging techniques results in improved sensitivity and specificity of both diagnostic and surveillance scans for children with medulloblastoma, neuroblastoma, and neuroendocrine tumors [58]. Castleberry et al. demonstrated how molecular targeting of somatostatin receptors can be exploited to differentiate embryonal versus astrocytic tumors at diagnosis and provide examples of the sensitivity of combined imaging techniques for identification of recurrent medulloblastoma.
They discuss the use of both MIBG and Octreoscan for molecularly targeted diagnoses and identification of metastatic disease in neuroblastoma and the new molecularly targeted imaging techniques including PET. The Authors also argue that the combination of imaging modalities is useful to identify early recurrences [59].
Complete surgery is the only known curative modality and unfortunately, more than half of NET (excluding appendiceal carcinoid) diagnosed in children are metastatic at diagnosis [2]. Although these tumors can produce distinct clinical syndromes due to their secretory capacity, they are under diagnosed in children, resulting in delays in detection [60].
A retrospective analysis of 30 patients (18 males 12 females; age range: 1-18 years; mean age 7.8 years) with histologically confirmed NETs underwent a 68Ga-DOTATATE PET/CT scan for primary staging. The completed study resulted in 11 neuroblastomas, 8 phaeochromocytomas, 5 GEP-NETs, 2 pancreatic NETs, 2 paragangliomas, 1 bronchial carcinoid and 1 ganglioneuroma. All of the above patients underwent a 68Ga-DOTATATE PET/CT scan at time of diagnosis for primary staging of their oncologic state.
Contrast enhanced CT performed at the time of PET scan acquisition was used for comparison with PET data. Eighteen-74 MBq of radioactivity of 68Ga-DOTATATE was injected intravenously in each patient and after 45-60 min 68Ga-DOTATATE PET/CT was performed. Diagnostic ceCT acquired with 68Ga-DOTATATE PET/CT scan was with 120 kV, 40 mA, in the age group of 0-3 years, 120 kV, 60 mA, in age group of 3-6 years and 120 kV, 70 mA, in age group of 6-12 years.
Seventeen/30 patients had no evidence of bone metastases on any imaging modality or on clinical follow-up while the rest of 13 patients showed evidence of bone metastases. Nine showed positivity both on 68Ga-DOTATATE PET and CT scan while four showed positivity only on 68Ga-DOTATATE PET. Compared with CT scan 68Ga-DOTATATE PET detected bone metastases at a significantly higher rate (P = 0.0039). On a per lesion analysis, out of a total of 225 lesions detected by 68Ga-DOTATATE PET, only 84 lesions could be detected by CT scan. Another study in a small patient cohort indicated that 68Ga-DOTA-TOC PET may be superior to 123I-MIBG scintigraphy and complementary to CT and MRI technique in providing particularly valuable information for pretherapeutic staging of phaeochromocytoma and neuroblastoma [61].
Conclusion
Several studies have shown the higher accuracy of 68Ga-DOTA-TOC for the detection of NET lesions as compared to conventional imaging. The use of 68Ga-DOTA-TOC is not only limited to a better overall accuracy but also provide valuable data regarding the pattern of expression of SST on target lesions, which represents a useful non-invasive modality for selecting patients for therapy with hot or cold somatostatin analogues. In response to treatment morphology-based criteria to assess tumor response have many limitations for NETs, which are often slow growing and frequently demonstrate low response rates when based on conventional radiological criteria, furthermore, many NET treatments do not induce cytotoxic effects and the lesion volume do not modify.
The imaging techniques which measure changes in tumor physiology and metabolism should be considered in treatment assessment and subsequently in the follow up. These include 68Ga-peptide PET/CT, molecular imaging with PET tracers that are not based on somatostatin receptor targeting and functional MRI. However, further data are needed to better plain the integrated use of metabolic and receptor-targeted tracers in the clinical management of patients with NET.
Conflict of interest
The authors confirm that this article content has no conflict of interest.
References
Key References: 5, 6, 8, 19, 21, 22, 23, 33, 34, 50, 53
- Arnold R, Chen YJ, Costa F, et al. ENETS Consensus Guidelines for the Standards of Care in Neuroendocrine Tumors: follow-up and documentation. Neuroendocrinology. 2009; 90(2): 227-233. [CrossRef] [PubMed Abstract]
- Modlin IM, Lye KD, Kidd M. A 5-decade analysis of 13,715 carcinoid tumors. Cancer. 2003; 97(4): 934-959. [CrossRef] [PubMed Abstract]
- Yao JC, Hassan M, Phan A, et al. One hundred years after ‘carcinoid’: epidemiology of and prognostic factors for neuroendocrine tumors in 35,825 cases in the United States. J Clin Oncol. 2008; 26(18): 3063-3072. [CrossRef] [PubMed Abstract]
- Ferolla P, Faggiano A, Mansueto G, et al. The biological characterization of neuroendocrine tumors: the role of neuroendocrine markers. J Endocrinol Invest. 2008; 31(3): 277-286. [CrossRef] [PubMed Abstract]
- Banerjee SR, Pomper MG. Clinical applications of Gallium-68. Appl Radiat Isot. 2013; 76: 2-13. [CrossRef] [PubMed Abstract]
- Reubi J.C. Somatostatin and other Peptide receptors as tools for tumor diagnosis and treatment. Neuroendocrinology. 2004; 80 (Suppl 1): 51-56. [CrossRef] [PubMed Abstract]
- Balon HR, Brown TL, Goldsmith SJ, et al. The SNM practice guideline for somatostatin receptor scintigraphy 2.0. J Nucl Med Technol. 2011; 39(4): 317-324. [CrossRef] [PubMed Abstract]
- Tang LH, Gonen M, Hedvat C, Modlin IM, Klimstra DS. Objective quantification of the Ki67 proliferative index in neuroendocrine tumors of the gastroenteropancreatic system: a comparison of digital image analysis with manual methods. Am J Surg Pathol. 2012; 36(12): 1761-1770. [CrossRef] [PubMed Abstract]
- Valente M, Catena L, Milione M, Pusceddu S, Formisano B, Bajetta E. Common Diagnostic Challenges in the Histopathologic Diagnosis of Neuroendocrine Lung Tumors: A Case Report. Case Rep Oncol. 2010; 3(2): 202-207. [CrossRef] [PubMed Abstract]
- Teng X.D. World Health Organization classification of tumours, pathology and genetics of tumours of the lung. Zhonghua Bing Li Xue Za Zhi. 2005; 34(8): 544-546. [PubMed Abstract]
- Rekhtman N. Neuroendocrine tumors of the lung: an update. Arch Pathol Lab Med. 2010; 134(11): 1628-1638. [PubMed Abstract]
- Bertino EM, Confer PD, Colonna JE, Ross P, Otterson GA. Pulmonary neuroendocrine/carcinoid tumors: a review article. Cancer. 2009; 115(19): 4434-4441. [CrossRef] [PubMed Abstract]
- Johnson R, Trocha S, McLawhorn M, et al. Histology, not lymph node involvement, predicts long-term survival in bronchopulmonary carcinoids. Am Surg. 2011; 77(12): 1669-1674. [PubMed Abstract]
- Fisseler-Eckhoff A, Demes M. Neuroendocrine tumors of the lung. Cancers (Basel). 2012; 4(3): 777-798. [CrossRef] [PubMed Abstract]
- Bradley JD, Dehdashti F, Mintun MA, Govindan R, Trinkaus K, Siegel BA. Positron emission tomography in limited-stage small-cell lung cancer: a prospective study. J Clin Oncol. 2004; 22(16): 3248-3254. [CrossRef] [PubMed Abstract]
- Shepherd FA, Crowley J, Van Houtte P, et al. The International Association for the Study of Lung Cancer lung cancer staging project: proposals regarding the clinical staging of small cell lung cancer in the forthcoming (seventh) edition of the tumor, node, metastasis classification for lung cancer. J Thorac Oncol. 2007; 2(12): 1067-1077. [CrossRef] [PubMed Abstract]
- Murray N Turrisi 3rd AT. A review of first-line treatment for small-cell lung cancer. J Thorac Oncol. 2006; 1(3): 270-278. [PubMed Abstract]
- Ozkara S Aker F, Yesil A, Senates E, Canbey C, Yitik A, et al. Re-evaluation of cases with gastroenteropancreatic neuroendocrine tumors between 2004 and 2012 according to the 2010 criteria. Hepatogastroenterology. 2013; 60(127): 1665-1672. [PubMed Abstract]
- Klimstra D.S. Pathology reporting of neuroendocrine tumors: essential elements for accurate diagnosis, classification, and staging. Semin Oncol. 2013; 40(1): 23-36. [CrossRef] [PubMed Abstract]
- Kunz PL, Reidy-Lagunes D, Anthony LB, et al. Consensus guidelines for the management and treatment of neuroendocrine tumors. Pancreas. 2013; 42(4): 557-577. [CrossRef] [PubMed Abstract]
- Mizutani G, Nakanishi Y, Watanabe N, et al. Expression of Somatostatin Receptor (SSTR) Subtypes (SSTR-1, 2A, 3, 4 and 5) in Neuroendocrine Tumors Using Real-time RT-PCR Method and Immunohistochemistry. Acta Histochem Cytochem. 2012; 45(3): 167-176. [CrossRef] [PubMed Abstract]
- Pape UF, Perren A, Niederle B, et al. ENETS Consensus Guidelines for the management of patients with neuroendocrine neoplasms from the jejuno-ileum and the appendix including goblet cell carcinomas. Neuroendocrinology. 2012; 95(2): 135-156. [CrossRef] [PubMed Abstract]
- Rockall AG, Reznek RH. Imaging of neuroendocrine tumours (CT/MR/US). Best Pract Res Clin Endocrinol Metab. 2007; 21(1): 43-68. [CrossRef] [PubMed Abstract]
- Hörsch D, Sayeg Y, Bonnet R, Kaemmerer D, Presselt N, Baum RP, et al. Expert dialogue: neuroendocrine tumours of the lungs and gastroenteropancreatic system. Pneumologie. 2012; 66(1): 44-48. [PubMed Abstract]
- Armbruster M, Zech CJ, Sourbron S, et al. Diagnostic accuracy of dynamic gadoxetic-acid-enhanced MRI and PET/CT compared in patients with liver metastases from neuroendocrine neoplasms. J Magn Reson Imaging. 2014; 40(2): 457-466. [CrossRef] [PubMed Abstract]
- Sommer WH, Zech CJ, Bamberg F, et al. Fluid-fluid level in hepatic metastases: a characteristic sign of metastases of neuroendocrine origin. Eur J Radiol. 2012; 81(9): 2127-3132. [CrossRef]
- Sankowski AJ, Ćwikla JB, Nowicki ML, et al. The clinical value of MRI using single-shot echoplanar DWI to identify liver involvement in patients with advanced gastroenteropancreatic-neuroendocrine tumors (GEP-NETs), compared to FSE T2 and FFE T1 weighted image after i.v. Gd-EOB-DTPA contrast enhancement. Med Sci Monit. 2012: 18(5): MT33-40. [CrossRef] [PubMed Abstract]
- d’Assignies G, Fina P, Bruno O, et al. High sensitivity of diffusion-weighted MR imaging for the detection of liver metastases from neuroendocrine tumors: comparison with T2-weighted and dynamic gadolinium-enhanced MR imaging. Radiology. 2013; 268(2): 390-399. [CrossRef] [PubMed Abstract]
- Gatto F, Hofland LJ. The role of somatostatin and dopamine D2 receptors in endocrine tumors. Endocr Relat Cancer. 2011; 18(6): R233-251. [CrossRef] [PubMed Abstract]
- Gouffon M, Iff S, Ziegler K, et al. Diagnosis and workup of 522 consecutive patients with neuroendocrine neoplasms in Switzerland. Swiss Med Wkly. 2014; 144: 13924. [CrossRef] [PubMed Abstract]
- Guidoccio F, Grosso M, Maccauro M, et al. Current role of 111In-DTPA-octreotide scintigraphy in diagnosis of thymic masses. Tumori. 2011; 97(2): 191-195. [PubMed Abstract]
- Sundin A, Rockall A. Therapeutic monitoring of gastroenteropancreatic neuroendocrine tumors: the challenges ahead. Neuroendocrinology. 2012; 96(4): 261-271. [CrossRef] [PubMed Abstract]
- Gabriel M, Decristoforo C, Kendler D, et al. 68Ga-DOTA-Tyr3-octreotide PET in neuroendocrine tumors: comparison with somatostatin receptor scintigraphy and CT. J Nucl Med. 2007; 48(4): 508-518. [CrossRef] [PubMed Abstract]
- Gabriel M, Oberauer A, Dobrozemsky G, et al. 68Ga-DOTA-Tyr3-octreotide PET for assessing response to somatostatin-receptor-mediated radionuclide therapy. J Nucl Med. 2009; 50(9): 1427-1434. [CrossRef] [PubMed Abstract]
- Sandström M, Velikyan I, Garske-Román U, et al. Comparative biodistribution and radiation dosimetry of 68Ga-DOTATOC and 68Ga-DOTATATE in patients with neuroendocrine tumors. J Nucl Med. 2013; 54(10): 1755-1759. [CrossRef] [PubMed Abstract]
- Prasad V, Baum RP. Biodistribution of the Ga-68 labeled somatostatin analogue DOTA-NOC in patients with neuroendocrine tumors: characterization of uptake in normal organs and tumor lesions. Q J Nucl Med Mol Imaging. 2010; 54(1): 61-67. [PubMed Abstract]
- Hofmann M, Maecke H, Börner R, et al. Biokinetics and imaging with the somatostatin receptor PET radioligand (68)Ga-DOTATOC: preliminary data. Eur J Nucl Med. 2001; 28(12): 1751-1757. [PubMed Abstract]
- Dromain C, de Baere T, Lumbroso J, et al. Detection of liver metastases from endocrine tumors: a prospective comparison of somatostatin receptor scintigraphy, computed tomography, and magnetic resonance imaging. J Clin Oncol. 2005; 23(1): 70-78. [CrossRef] [PubMed Abstract]
- Henze M, Schuhmacher J, Hipp P, et al. PET imaging of somatostatin receptors using [68GA]DOTA-D-Phe1-Tyr3-octreotide: first results in patients with meningiomas. J Nucl Med. 2001; 42(7): 1053-1056. [PubMed Abstract]
- Henze M, Dimitrakopoulou-Strauss A, Milker-Zabel S, et al. Characterization of 68Ga-DOTA-D-Phe1-Tyr3-octreotide kinetics in patients with meningiomas. J Nucl Med. 2005; 46(5): 763-769. [PubMed Abstract]
- Kowalski J, Henze M, Schuhmacher J, Mäcke HR, Hofmann M, Haberkorn U, et al. Evaluation of positron emission tomography imaging using [68Ga]-DOTA-D Phe(1)-Tyr(3)-Octreotide in comparison to [111In]-DTPAOC SPECT. First results in patients with neuroendocrine tumors. Mol Imaging Biol. 2003; 5(1): 42-48. [PubMed Abstract]
- Buchmann I, Henze M, Engelbrecht S, et al. Comparison of 68Ga-DOTATOC PET and 111In-DTPAOC (Octreoscan) SPECT in patients with neuroendocrine tumours. Eur J Nucl Med Mol Imaging. 2007; 34(10): 1617-1626. [CrossRef] [PubMed Abstract]
- Pettinato C, Sarnelli A, Di Donna M, et al. 68Ga-DOTANOC: biodistribution and dosimetry in patients affected by neuroendocrine tumors. Eur J Nucl Med Mol Imaging. 2008; 35(1): 72-79. [CrossRef] [PubMed Abstract]
- Fanti S, Ambrosini V, Tomassetti P, et al. Evaluation of unusual neuroendocrine tumours by means of 68Ga-DOTA-NOC PET. Biomed Pharmacother. 2008; 62(10): 667-671. [CrossRef] [PubMed Abstract]
- Ambrosini V, Rubello D, Nanni C, Al-Nahhas A, Fanti S. 68Ga-DOTA-peptides versus 18F-DOPA PET for the assessment of NET patients. Nucl Med Commun. 2008; 29(5): 415-417. [CrossRef] [PubMed Abstract]
- Prasad V, Ambrosini V, Hommann M, Hoersch D, Fanti S, Baum RP. Detection of unknown primary neuroendocrine tumours (CUP-NET) using (68)Ga-DOTA-NOC receptor PET/CT. Eur J Nucl Med Mol Imaging. 2010; 37(1): 67-77. [PubMed Abstract]
- Garin E, Le Jeune F, Devillers A, et al. Predictive value of 18F-FDG PET and somatostatin receptor scintigraphy in patients with metastatic endocrine tumors. J Nucl Med. 2009; 50(6): 858-864. [CrossRef] [PubMed Abstract]
- Naswa N, Sharma P, Gupta SK, et al. Dual tracer functional imaging of gastroenteropancreatic neuroendocrine tumors using 68Ga-DOTA-NOC PET-CT and 18F-FDG PET-CT: competitive or complimentary? Clin Nucl Med. 2014; 39(1): e27-34. [CrossRef] [PubMed Abstract]
- Virgolini I, Ambrosini V, Bomanji JB, et al. Procedure guidelines for PET/CT tumour imaging with 68Ga-DOTA-conjugated peptides: 68Ga-DOTA-TOC, 68Ga-DOTA-NOC, 68Ga-DOTA-TATE. Eur J Nucl Med Mol Imaging. 2010; 37(10): 2004-2010. [CrossRef] [PubMed Abstract]
- Ambrosini V, Fanti, S. 68Ga-DOTA-peptides in the diagnosis of NET. PET Clin. 2014. 9(1): 37-42. [CrossRef] [PubMed Abstract]
- Sharma P, Arora S, Mukherjee A, et al. Predictive value of 68Ga-DOTANOC PET/CT in patients with suspicion of neuroendocrine tumors: is its routine use justified? Clin Nucl Med. 2014; 39(1): 37-43. [CrossRef] [PubMed Abstract]
- Naswa N, Sharma P, Soundararajan R, et al. Diagnostic performance of somatostatin receptor PET/CT using 68Ga-DOTANOC in gastrinoma patients with negative or equivocal CT findings. Abdom Imaging. 2013; 38(3): 552-560. [CrossRef] [PubMed Abstract]
- Haug AR, Cindea-Drimus R, Auernhammer CJ, et al. Neuroendocrine tumor recurrence: diagnosis with 68Ga-DOTATATE PET/CT. Radiology. 2014; 270(2): 517-525. [PubMed Abstract]
- Castaño JP, Sundin A, Maecke HR, et al. Gastrointestinal neuroendocrine tumors (NETs): new diagnostic and therapeutic challenges. Cancer Metastasis Rev. 2014; 33(1): 353-359. [PubMed Abstract]
- Schiesser M, Veit-Haibach P, Muller MK, et al. Value of combined 6-[18F]fluorodihydroxyphenylalanine PET/CT for imaging of neuroendocrine tumours. Br J Surg. 2010; 97(5): 691-697. [CrossRef] [PubMed Abstract]
- Kauhanen S, Seppänen M, Ovaska J, et al. The clinical value of [18F]fluoro-dihydroxyphenylalanine positron emission tomography in primary diagnosis, staging, and restaging of neuroendocrine tumors. Endocr Relat Cancer. 2009; 16(1): 255-265. [CrossRef] [PubMed Abstract]
- Delle Fave G, Kwekkeboom DJ, Van Cutsem E, et al. ENETS Consensus Guidelines for the management of patients with gastroduodenal neoplasms. Neuroendocrinology. 2012; 95(2): 74-87. [CrossRef] [PubMed Abstract]
- Gaal J, de Krijger RR. Neuroendocrine tumors and tumor syndromes in childhood. Pediatr Dev Pathol. 2010; 13(6): 427-441. [CrossRef] [PubMed Abstract]
- Castleberry RP. Neuroblastoma. Eur J Cancer. 1997; 33(9): 1430-1437. [CrossRef] [PubMed Abstract]
- Khanna G, O’Dorisio SM, Menda Y, Kirby P, Kao S, Sato Y. Gastroenteropancreatic neuroendocrine tumors in children and young adults. Pediatr Radiol. 2008; 38(3): 251-259. [CrossRef] [PubMed Abstract]
- Kroiss A, Putzer D, Uprimny C, et al. Functional imaging in phaeochromocytoma and neuroblastoma with 68Ga-DOTA-Tyr 3-octreotide positron emission tomography and 123I-metaiodobenzylguanidine. Eur J Nucl Med Mol Imaging, 2011; 38(5): 865-873. [PubMed Abstract]
Article information
Corresponding author: Elisabetta Giovannini.
Copyright: © 2014 Giovannini E et al. This is an open-access article distributed under the terms of the Creative Commons Attribution License, which permits unrestricted use, distribution, and reproduction in any medium, provided the original author and source are cited.
How to cite: Giovannini E, Gaeta M, Ciarmiello A. 68Ga-Somatostatin analogue PET/CT in neuroendocrine tumors. J. Diagn Imaging Ther. 2014; 1(1): 81-102 (https://dx.doi.org/10.17229/jdit.2014-1030-006).
Article history: Received 07 October 2014; Revised 25 October 2014; Accepted: 29 October 2014; Published online 30 October 2014.
Archive link: JDIT-2014-1030-006
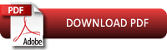
You are here: home »