Clinical radiology uses several medical imaging techniques, including radiopharmaceuticals, X-rays, computed tomography CT, and radiography.
Wilhelm Conrad Röntgen produced the first radiographic images in 1895. Today, clinical radiology is a medical specialty that uses an array of imaging techniques to help in the patient’s overall diagnosis. The radiologists’ toolbox consists of various modern imaging modalities exemplified by computed tomography CT, amongst others. Another technique uses acoustics, creating 3-D images in real time without the need for radiation: this is called ultrasonography.
Magnetic resonance imaging (MRI), however, makes use of powerful magnetic fields (>3 teslas) and radiofrequency pulses to generate 2-D and 3-D (axial, coronal, sagittal) images without the use of radiation. Other clinical radiology modes of scanning include functional MRI imaging, cardiovascular MRI and MRI-guided therapy.
Nuclear Medicine produces images by administering short-lived radiopharmaceuticals to the patient. The radiation released by the patient can be detected using a gamma camera or positron emission scanner to enable the overall image. Nuclear Medicine radiology modalities employed in the clinical setting are single photon emission computed tomography (SPECT), positron emission tomography (PET) and hybrids combining computed tomography (CT) and magnetic resonance imaging (MRI) scanners.
Diagnostic Imaging in Clinical Radiology: Radiography, CT, MRI, and Beyond
Radiography is a medical imaging technique that utilises X-rays to view internal structures in the human body. X-ray beams are generated and directed at the patient. These X-rays pass through the body, are detected, and then processed to obtain the image. The detector provides a superimposed 2-D representation of all the body’s internal structures. The advancement of radiography has enabled the principle of tomography. In this approach, the X-ray source and detector move to obscure structures that are not in the focal plane. Unlike plain film tomography, computed tomography (CT) generates 3-D representations used for computer-assisted reconstruction. The radiation dosages in this imaging process range from 3 mSv to 20 mSv, depending on the procedure. Today, the role of radiography has expanded dramatically as a result of more advanced equipment for radiography.
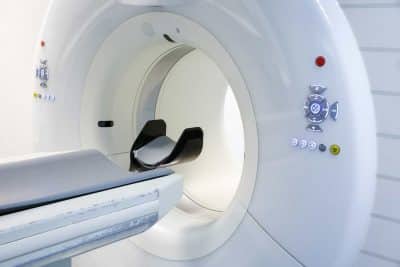
PET Imaging with Radiopharmaceutical Agents: Molecular Imaging in Clinical Practice
Radiopharmaceuticals are radioactive drugs and/or imaging agents containing a radionuclide (radioisotope). Several radiotracers have been given FDA approval in the clinical setting for radiology diagnosis (e.g. fluorine-18 fludeoxyglucose, ProstaScint®, Octreoscan®, DaTscan™) and/or therapy (e.g. Zevalin®, Xofigo®, Bexxar®). Medical physicists calculate the dosimetry of the radiopharmaceutical to enable safe injection into the patient’s systemic circulation. Occasionally, the radiopharmaceutical can be given orally, and this depends on the disease state and the imaging technique used.
Radiopharmaceuticals used for diagnosis contain an unstable radionuclide that decays by emitting electromagnetic radiation. The dose is usually in the form of gamma or röentgen radiation. During a typical decay process, positrons are released, which are capable of interacting with electrons. This electron-positron annihilation process generates two gamma rays of 511 keV in opposite directions.
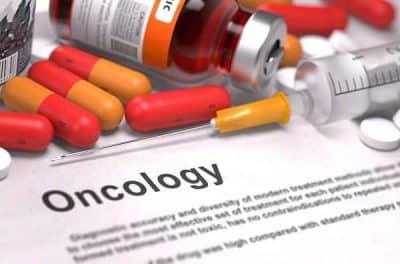
An important property of gamma radiation is its high penetrative power, capable of being absorbed partially by tissues in the human body. When a patient receives a diagnostic radiopharmaceutical, the resultant gamma or X-ray radiation can be detected externally from the body by using gamma or positron emission tomography (PET) cameras.
Radiopharmaceuticals, labelled with the radionuclides carbon-11 (t½= 20.4 mins), nitrogen-13 (t½= 10 mins), oxygen-15 (t½= 2.07 mins) and fluorine-18 (t½= 110 mins), are all employed in PET imaging due to their short half-life. Consequently, they must be prepared near the patient. Methods have also been developed to generate the radionuclide by using radionuclide generators (e.g. Drytec™) which prepare the gamma emitter technetium-99m (t½= 6 hours) labelled radiopharmaceuticals in the form of disposable labelling kits (Myoview™).
The continuing advancement of radiosynthesis technologies, especially in the area of automation rigs (FASTlab2) is establishing a broad range of radiopharmaceuticals to be used in the clinical setting. Currently, radiotherapeutics are of significant interest due to the approval of the first alpha particle-emitting therapeutic, Xofigo®. Further developments in this sphere lead to novel beta-gamma radiation based on medicinal drugs and/or radio-imaging agents.
Radiotherapeutics in Oncology: Combining Imaging and Treatment for Improved Outcomes
The therapeutic nature of ionising radiation is useful in medical imaging by applying X-rays to kill tumour cells for the different stages of cancer. These radiopharmaceuticals contain a therapeutic radionuclide, which decays by emitting beta particles. Beta particles are fast, energetic electrons projecting over short distances by transporting their energy to a defined target volume resulting in killing tumour cells or altering the cell function.
Consequently, radiotherapeutics designed in sealed sources (cobalt-60, radioactive seeds) are known as Brachytherapy or internal radiotherapy. These devices are placed strategically in the human body, so the emitted radiation kills the tumour. In addition to surgery, Brachytherapy is used in conjunction with other therapies, such as external beam radiotherapy (EBRT) and chemotherapy.
Another important approach is to use radionuclide antibody conjugates (RACs) to target cancer in a specific part of the body by firing the radiation in the form of alpha (targeted alpha radiotherapy) and beta particles to kill cancer cells. This approach depends on the radionuclide’s linear energy transfer (LET).
A range of radionuclides (actinium-225, bismuth-213, astatine-211) is capable of emitting short-range particles such as alpha or beta. This property is beneficial in therapy because it can irradiate the energy over short distances and thus limit the damage to surrounding healthy cells.
Several therapeutic radioisotopes survive longer in the human body because the objective is to increase the efficiency of the treatment. These radionuclides used in the therapeutic mode are iodine-131 (Bexxar®), yttrium-90 (Zevalin®), rhenium-188 and lutetium-177. The emitted radiation used in medical procedures eventually decays in a reasonable time for the patient to leave the hospital.
You are here: home »