RESEARCH ARTICLE
Fagner Santos do Carmo1, Suyenne Rocha Pinto1, Margarida Maria Camões Orlando2, Marta de Souza Albernaz3, Mara de Souza Junqueira4, Emerson Soares Bernardes4, Hugo Cerecetto5, Albertina Moglioni6, Jan Kozempel7, Marzena Szwed8, Sotiris Missailidis9, Ralph Santos-Oliveira1,*
1 Zona Oeste State University, Laboratory of Nano-Radiopharmaceuticals and Radiopharmacy, Brazil
2 Rio de Janeiro State University, University Hospital Pedro Ernesto, Brazil
3 Federal University of Rio de Janeiro, University Hospital Clementino Fraga Filho, Brazil
4 Laboratório de Oncologia Experimental, Faculdade de Medicina, Universidade de São Paulo, Brazil
5 University of the Republic, Centro de Investigaciones Nucleares, Uruguay
6 Universidad de Buenos Aires, Facultad de Farmacia y Bioquimica, Argentina
7 Czech Technical University in Prague, Faculty of Nuclear Sciences and Physical Engineering, Czech Republic
8 University of Lodz, Institute of Biophysics, Lodz, Poland
9 Federal University of Rio de Janeiro, Institute of Biophysics, Brazil
Abstract
The application of aptamers especially in the use of drug delivery systems (DDSs) has the potential to develop in vivo nanoparticles for theranosis (therapy+diagnosis). With the advent of medical imaging and radiotherapeutics, this area of research developing the next era of radiopharmaceuticals is both attractive and promising. Overall, nano-radiopharmaceuticals have the potential to solve several problems regarding the in vivo stability of aptamers. This paper discusses a study in the development and proof-of-concept of nano-aptamers and supporting its use as a nano-radiopharmaceutical for the treatment of breast cancer and other potentially related disease states.
Keywords: radiopharmacy; radiopharmaceuticals; cancer; oncology; drug delivery systems; nano-aptamer
1. Introduction
In general, the main principle of a drug delivery system (DDS) is the ability of the carrier for a tumour to recognize the tumour part of the DDS. The conjugate acting as a pro-drug should be non-toxic and stable in the systemic circulation. As soon as the conjugate is internalized into the tumour cell, the DDS must quickly release the cytotoxic drug to be effective. Similarly, the radiopharmaceutical linked to the tumour cell must be given adequate time for the DDS to emit the radiation dose and image the tumour cells [1–3].
It is essential that the DDS must be able to differentiate between healthy and abnormal tissue types and crucial that the DDS recognizes the morphological and physiological states of healthy and diseased tissue types. Therefore the mechanism of tumour tissue can be used to enter the tumour site. Tumour site tissue proliferates rapidly and consequently leads to the formation of the newly defective vasculature. The DDS carriers allow large molecules and lipids to penetrate more effectively into the extravascular space. Conversely, lymphatic drainage is poor and therefore large molecules and lipids will not be able to escape easily from the tumour site. This effect is known as enhanced permeability and retention (EPR). The accumulation of macromolecules, including plasma proteins, does not require a specific receptor. Therefore, the mechanism of EPR probably acts as a passive transport system within the cells [1–3].
The main physiological characteristic of tumour cells is a high metabolic rate causing hypoxia. Anaerobic metabolism is induced resulting in the formation of lactate, which lowers intracellular pH [3–4]. For this reason, several DDSs/carriers are designed to release the drug at an acidic pH. Moreover, with its rapid growth, tumours require various nutrients and vitamins in order to overexpress a variety of tumour-specific receptors that are potential targets for DDSs radiopharmaceuticals. Therefore, various molecules can be conjugated to DDSs/carriers to serve as drivers for the tumour. These include monoclonal antibodies, polyunsaturated fatty acids, folic acid, hyaluronic acid, oligopeptides and aptamers [4–7].
The foremost limitation concerning aptamer technology regards in vivo theranosis. The use of which has been susceptible to degradation by endo- and exonucleases and their pharmacokinetic properties [8]. In this respect, incorporating the aptamer into polymeric nano-particles is expected to provide increased stability and protection from nuclease degradation. In addition, the pharmacokinetic profile and cell internalization properties are improved and developed and tested in this current study [8,9].
The aptamers used in this study have been well-characterized against the tumour marker MUC1. These aptamers, directed against the protein core of MUC1 and the glycosylated form of the protein including the Tn antigen, are produced by the group of Missailidis in the UK. Furthermore, they are proving to be valuable reagents in diagnostic imaging and photodynamic therapy [9] against several breast cancer models.
Significantly, these aptamers were utilized further in several studies to demonstrate their incorporation into polymeric nanoparticles. Furthermore, this opens up the possibility for future study in developing cancer models [5,10–19].
2. Methodology
2.1 Nanoparticles
The PLA/aptamer nanoparticles have been prepared according to the technique of single-dye-doped silica nanoparticles. These nanoparticles were obtained by using 1 mL of the aqueous solution formed by 1% PVA with 5 mg of aptamer (anti-MUC-1). This matrix was allowed to drip into a cold solution containing 120 mg of PLA dissolved in 2 ml of dichloromethane, then sonicated for 2 min to form an emulsion (water in oil, W/O). The resultant emulsion was then evaporated under reduced pressure at a temperature of 35 °C to eliminate the residual solvent. Subsequently, the nanoparticles were centrifuged (7000 rpm) and washed with distilled water.
2.2 Labelling with 99mTc-Nano-Radiopharmaceuticals
The process of labelling PLA/aptamer was by the direct method using the radionuclide 99mTc as described in [20–22].
3. In vivo analysis
3.1 Tumour xenograft models
Female Balb/c nude mice at 8-9 weeks of age were inoculated subcutaneously with 100 μL of cell suspension MDA-MB-231 (1 × 106 cells), in the right flank. Tumour growth was monitored twice each week, by measuring the tumour size using calipers.
3.2 Biodistribution
The biodistribution studies were completed by utilizing a single nude mouse. The Institutional Review Board and the Animal Ethics Committee approved the study protocol. The labelled samples, approximately 3.7 MBq/0.3 mL were intraocularly administered into the mice. The counts were acquired at a 5 min interval, in a 15% window, centred at 140 keV.
4. Cytotoxicity
4.1 Cell cultures
The cell lines MCF-7 (human breast adenocarcinoma non-expressed estrogen receptors) and MDA-MB 231 (human breast adenocarcinoma expressed estrogen receptors), were grown in monolayer in the presence of Dulbecco’s Modified Eagle Medium (DMEM). This medium contained glucose (4.5 g/L) and supplemented with 10% heat-inactivated fetal bovine serum, 1 mM of L-glutamine and 1 mM of sodium pyruvate.
The Neuro-2a and SKOV-3 cells were cultured in monolayer using the RPMI 1640 medium, containing 10% heat-inactivated fetal bovine serum. These cells were kept in a water saturated incubator at 37 °C with an atmosphere containing 5% CO2 and maintained in the exponential growth phase by routine subculturing every 2-3 days. These cells were monitored periodically to ensure they were mycoplasma free.
4.2 Cell cytotoxicity assay
The cytotoxicity of EDTMP, aptamer and PLA nanoparticles on human tumour cells were measured using 96-well plates by an MTT [1-(4,5-dimethylthiazol-2-yl)-3,5-diphenylformazan, thiazolyl blue formazan] colorimetric assay.
This method which is based on the cleavage of MTT by metabolically active cells. For this purpose, 104 cells were seeded in each well in 0.1 mL of culture medium. In the next step 0.05 mL of EDTMP, PLA and aptamer nanoparticles of different concentrations were added to the appropriate wells. The above cells were incubated with drugs for 72 h. At the end of incubation, 50 μL MTT at a final concentration of 0.05 mg/mL HBSS (140 mM NaCl, 5 mM KCl, 0.8 mM MgCl2, 1.8 mM CaCl2, 1 mM Na2HPO4, 10 mM HEPES, and 1% glucose) were added to each well and the microplates were incubated for 4 h. Subsequently, the formazan crystals were dissolved in DMSO (dimethyl sulfoxide), and plates were mechanically agitated for 1 min. An absorbance wavelength at 580 nm was measured by using a microplate reader (Awareness Technology Inc., USA).
4.3 Statistical analysis
This experimental data was obtained by the mean of standard deviation (± S.D.). The analysis of variance (ANOVA) was completed with the aid of a Tukey post hoc test which is utilised for multiple comparisons. All statistics were obtained by using the STATISTICA program (StatSoft, Tulsa, OK, USA) where a resultant p value of < 0.05 was considered significant.
5. Results and discussion
Many cancer treatments involve the traditional approach of using chemotherapy and radiotherapy. The major disadvantage of these therapies is due to the toxic damage to the surrounding healthy tissue. To limit the damage to these cells, we have developed an experimental ‘personalized’ approach to the treatment of breast cancer. This targeted approach using nano-based drug delivery systems (DDSs) offers a possible alternative to the use of monoclonal antibody-based drugs such as antibody-drug conjugates (ADCs) and radionuclide-antibody conjugates (RACs). The advantage of using nano-particles is the possibility of fewer side-effects owing to immunogenicity and the reduced cost of the commercial production of designer antibodies. These studies have shown several advantages of using aptamer technology over antibody therapies and the nano-aptamer demonstrated a size of between 100-200 nm and was spherical in shape (Figure 1). This nano-aptamer size is particularly favourable for medical application because it allows the usage of several imaging modalities such as SPECT and CT imaging.
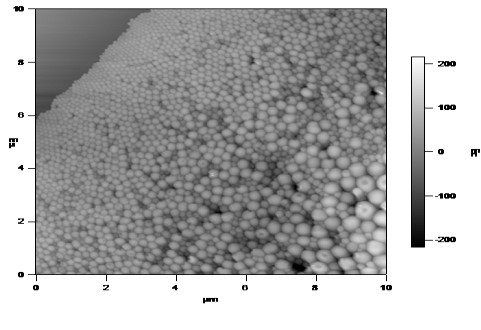
.
Figure 1. Nano-aptamer with a size variation between 100-200 nm.
5.1 Cytotoxicity
To determine the survival of both normal and cancer cells after nano-aptamer treatment, we utilised the spectrophotometric method, in conjunction with XTT. The graphs below (Figure 2) show the comparison of the nanoparticle towards various cell types. In the case of MDA-MB 231 and MCF-7, it was noticed that the nano-aptamer at a dose of 500 μg/mL decreased cell survival to 80%. However, the aim of this study is to demonstrate that the nano-aptamer is capable of killing tumour cells our data also supports the migration of the nanoparticle to the particular site of the tumour. These observations were confirmed by scintigraphic imaging.
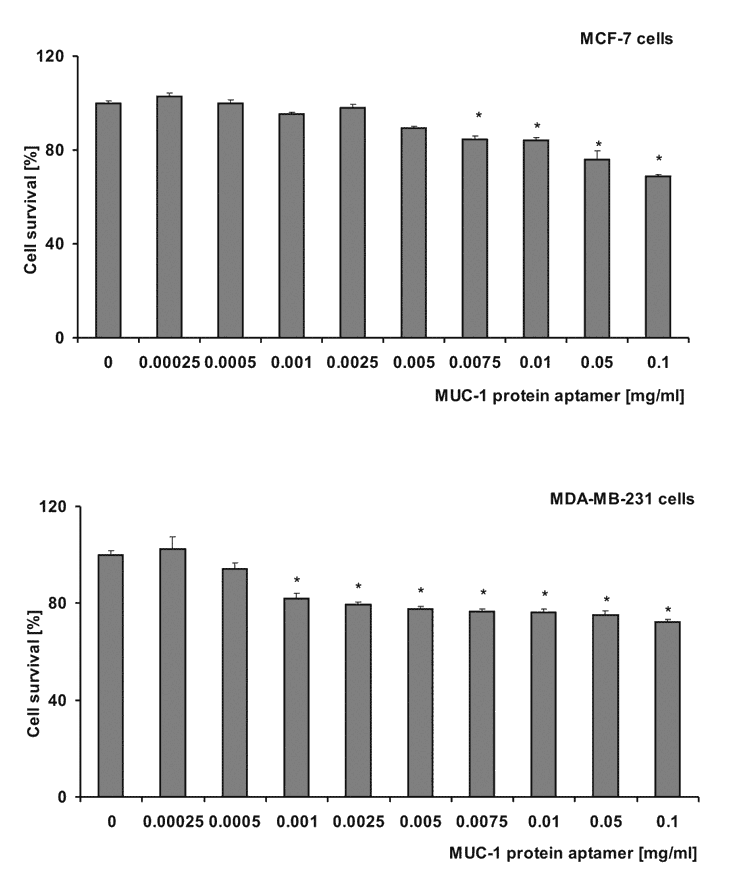
Figure 2. The effect of aptamer nanoparticles on the survival of A549, MCF-7, MDA-MB 231, Neuro-2a and SKOV-3 cells. The cells in RPMI/DMEM underwent treatment with various concentrations of nanoparticles for 72 h. The survival rate was estimated using the MTT assay with measured values (mean ± SD; n ≥ 3). These were calculated as a percentage of those obtained from the untreated control (*p < 0.05).
5.2 Biodistribution
Figure 3 indicates the onset of the nano-particles biodistribution to breast cancer; this is located on the flank of the animal and invisible to the migration site. Although all the necessary precautions were taken regarding the welfare of the mice, a number of them eventually died during the imaging process. This unfortunate limitation was resultant in hampering the imaging process.
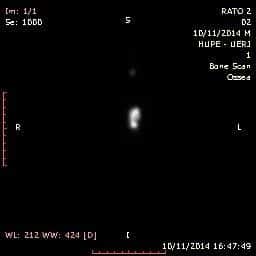
Figure 3. Scintigraphy of nano-aptamers in the breast cancer animal model. The methodology to obtain nano-aptamers does appear to be quite attractive. The usage of aptamers as nanoparticles is promising, as one of the major problems related to aptamers is the rapid efficacy-loss due to the tumour microenvironment, especially the pH. The aptamers seem not to exist encapsulated and therefore long-circulating aptamers were present.
6. Conclusion
In this study, we have demonstrated the application of an aptamer nano-particle to image breast tumours in mice. Furthermore, it is important to realize that the encapsulation of the aptamer does not interfere with the position of the tumour site. In the future, this opens new possibilities for the design and development of innovative nano-radiopharmaceuticals for diagnostic imaging and treatment plans.
Conflict of interest
The authors confirm that this article content has no conflict of interest.
References
Key References: 1, 2, 11, 13, 21
- Itakura S, Hama S, Ohgita T, Kogure K. Development of nano-particles incorporating a novel liposomal membrane destabilization peptide for efficient release of cargos into cancer cells. PLoS One. 2014; 9(10): e111181.[CrossRef] [PubMed Abstract]
- Makino A, Kimura S. Solid tumor-targeting theranostic polymer nano-particle in nuclear medicinal fields. ScientificWorldJournal. 2014; 2014: 424513.[CrossRef] [PubMed Abstract]
- Sakurai Y, Kajimoto K, Hatakeyama H, Harashima H. Advances in an active and passive targeting to tumor and adipose tissues. Expert Opin Drug Deliv. 2015; 12(1): 41-52.[CrossRef] [PubMed Abstract]
- Kong RM, Ding L, Wang Z, You J, Qu F. A novel aptamer-functionalized MoS2 nanosheet fluorescent biosensor for sensitive detection of prostate specific antigen. Anal Bioanal Chem. 2015; 407(2): 369-377.[CrossRef] [PubMed Abstract]
- de Franciscis V. A theranostic “SMART” aptamer for targeted therapy of prostate cancer. Mol Ther. 2014; 22(11):1886-1888.[CrossRef] [PubMed Abstract]
- Le TT, Adamiak B, Benton DJ, et al. Aptamer-based biosensors for the rapid visual detection of flu viruses. Chem Commun (Camb). 2014; 50(98): 15533-15536.[CrossRef] [PubMed Abstract]
- Sá LT, Pessoa C, Meira AS, da Silva MI, Missailidis S, Santos-Oliveira R. Development of nanoaptamers using a mesoporous silica model labeled with (99m)tc for cancer targeting. Oncology. 2012; 82(4): 213-217.[Reference Source] [PubMed Abstract]
- Ara MN, Matsuda T, Hyodo M, et al. Construction of an aptamer modified liposomal system targeted to tumour endothelial cells. Biol Pharm Bull. 2014; 37(11): 1742-1749.[CrossRef] [PubMed Abstract]
- Manandhar Y, Bahadur KC, Wang W, Uzawa T, Aigaki T, Ito Y. In vitro selection of a peptide aptamer that changes fluorescence in response to verotoxin. Biotechnol Lett. 2015; 37(3): 619-625.[CrossRef] [PubMed Abstract]
- Zhou C, Chen T, Wu C, et al. Aptamer CaCO3 nanostructures: a facile, pH-responsive, specific platform for targeted anticancer theranostics. Chem Asian J. 2015; 10(1): 166-171.[Reference Source] [PubMed Abstract]
- Sá LT, Simmons S, Missailidis S, da Silva MI, Santos-Oliveira R. Aptamer-based nanoparticles for cancer targeting. J Drug Target. 2013; 21(5): 427-434.[Reference Source] [PubMed Abstract]
- Niazi JH, Verma SK, Niazi S, Qureshi A. In vitro HER2 protein-induced affinity dissociation of carbon nanotube-wrapped anti-HER2 aptamers for HER2 protein detection. Analyst. 2015; 140(1): 243-249.[CrossRef] [PubMed Abstract]
- Perkins A, Missailidis S. Radiolabelled aptamers for tumour imaging and therapy. Q J Nucl Med Mol Imaging. 2007; 51(4): 292-296.[Reference Source] [PubMed Abstract]
- Borbas KE, Ferreira CSM, Perkins A, Bruce JI, Missailidis S. Design and synthesis of mono- and multimeric targeted radiopharmaceuticals based on novel cyclen ligands coupled to anti-MUC1 aptamers for the diagnostic imaging and targeted radiotherapy of cancer. Bioconjugate Chem. 2007; 18(4): 1205-1212.[CrossRef]
- Missailidis S, Perkins A. Aptamers as novel radiopharmaceuticals: Their applications and future prospects in diagnosis and therapy. Cancer Biother Radiopharm. 2007; 22(4): 453-468.[Reference Source] [PubMed Abstract]
- Ferreira CSM, Cheung MC, Missailidis S, Bisland S, Gariépy J. Phototoxic aptamers selectively enter and kill epithelial cancer cells. Nucleic Acids Res. 2009; 37(3): 866–876.[CrossRef] [PubMed Abstract]
- Ferreira CSM, Papamichael K, Guilbault, G, Schwarzacher T, Gariepy J, Missailidis S. DNA aptamers against MUC1: Design of aptamer-antibody sandwich ELISA for early tumour diagnosis. Anal Bioanal Chem. 2008; 390(4): 1039-1050.[Reference Source] [PubMed Abstract]
- DaPieve C, Perkins AC, Missailidis S. Anti-MUC1 aptamers: radiolabelling with 99mTc and biodistribution in MCF-7 tumour-bearing mice. Nuclear Med Biol. 2009; 36: 703-710.[Reference Source] [PubMed Abstract]
- Leite Diniz C, Da Pieve C, Perkins A, et al. 1.227 Pharmacokinetic and biodistribution studies of anti MUC1 PEGylated aptamers with potential in the targeted radiotherapy of breast cancer. EJC Supplements. 2010; 8(5): 59-60.[CrossRef]
- Patricio BFC, Weissmuller G, Santos-Oliveira R. Development of the 153-SM-EDTMP nanoradiopharmaceutical. J Label Compd Radiopharm. 2011; 54: S558.[Reference Source]
- Patricio BFC, Albernaz MS, Sarcinelli MA, de Carvalho SM, Santos-Oliveira R, Weissmüller G. Development of novel nanoparticle for bone cancer. J Biomed Nanotechnol. 2014; 10(7): 1242-1248.[CrossRef] [PubMed Abstract]
- Pires J, Patrico BFC, Albernaz MS, et al. Preparation of biodegradable poly(L-Lactide) (PLA) nanoparticles containing DMSA (dimercaptosuccinic acid) as novel radiopharmaceutical. Advanced Science Letters. 2012; 10(1): 143-145.[CrossRef]
Article information
Corresponding author: Ralph Santos-Oliveira.
Copyright: © 2015 Santos do Carmo F et al. This is an open-access article distributed under the terms of the Creative Commons Attribution License, which permits unrestricted use, distribution, and reproduction in any medium, provided the original author and source are cited.
How to cite: Santos do Carmo F, Rocha Pinto S, Maria Camões Orlando M, et al. Nano-Aptamer for Breast Cancer Imaging: Initial Considerations. Journal of Diagnostic Imaging in Therapy. 2015; 2(2): 41-49 (https://dx.doi.org/10.17229/jdit.2015-0518-018).
Article history: Received: 07 May 2015; revised: 15 May 2015; Accepted: 16 May 2015; Published online 18 May 2015.
Archive link: JDIT-2015-0518-018
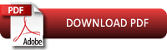
You are here: home »