- Technetium-99m generator
- How do radiopharmaceuticals work?
- SPECT imaging
- Commonly used SPECT radiometals
- PET imaging
- PET radiometals
- Molecular imaging capabilities of PET
- Beta particles
- Alpha particles
- Electron capture
- Therapy radiometals
- Criteria for the ideal radiometal
- Radiometal chelate properties:
- Antibodies
- Zevalin - better together
- Peptides
- Imaging and therapy radiometal table
- Conclusion
Imaging agents can be used to evaluate organ function, detect cancer, measure blood flow and follow metabolic processes.
Technetium-99m generator
The first radiometal molybdenum-99/technetium-99m generator was developed at the Brookhaven National Laboratory in 1959. Then in 1964, technetium-99m radiotracers were being established at the Argonne National Laboratory. Currently, 85% of radiopharmaceuticals use technetium-99m based imaging agents. These imaging agents can be used to evaluate organ function, detect cancer, measure blood flow and follow metabolic processes.
The Imaging Periodic Table below gives an overview of the types of radiation emitted from various radionuclides.
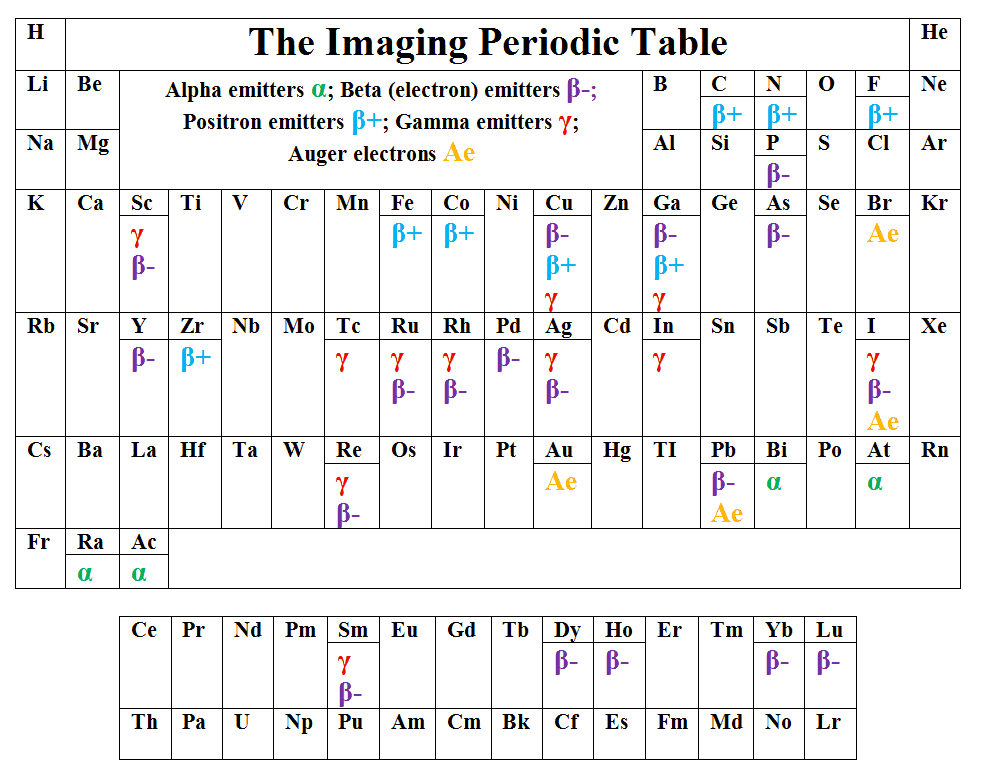
Imaging Periodic Table
How do radiopharmaceuticals work?
When a radiopharmaceutical enters the body, it concentrates in organs and tissues and is therefore dependent on the biological or physiological characteristics of the tissue. For example, it may be concentrated at the site of a tumour or infection, or in a particular organ. The patient can then be scanned to image the radioactivity and this gives an overall picture of what is happening in the body.
An advantage of this method over other imaging techniques is that the dose of the radioactive compound can be given in nanomolar quantities or less. This contrasts with other technologies such as magnetic resonance imaging where gadolinium-containing agents are used to enhance the image by using millimolar amounts of the agent to administer to the patient.
SPECT imaging
There is a broad range of radiometals that are used for cancer theranostics: these process a different mode of radioactive decay; therefore making them suitable for particular applications. The majority of diagnostic tools use radiometals, which emit gamma rays and are detected using a gamma camera. This captures the rays with a sodium iodide crystal, amplifies the signal and uses it to create an image. The image can be in a single plane parallel to the gamma camera, or a three-dimensional projection which can be built up using a computer-assisted reconstruction technique known as single photon emission computed tomography (SPECT).
SPECT imaging uses radionuclides that emit a single photon of energy and these include the following gamma-ray emitters.
Commonly used SPECT radiometals
Molecular Imaging Capabilities of SPECT
- uncovering, staging and monitoring various cancers.
- examining deep venous thrombosis.
- measuring multi-drug resistance to chemotherapy.
- imaging angiogenesis and apoptosis for early diagnoses and measures of therapeutic response.
- diagnosing and evaluating Parkinson’s disease and other neurodegenerative conditions.
PET imaging
A second common imaging technique found in nuclear medicine departments is positron emission tomography (PET). PET imaging uses radionuclides which emit a positron (a positively charged particle with the same mass as an electron) from the nucleus. This positron collides with an electron and both particles are annihilated. During this process, 511 KeV of energy is released in the form of two gamma rays which travel in opposite directions.
A series of gamma detectors are placed around the patient, allowing both the location and amount of radioactivity in the patient to be calculated: this eventually leads to a processed image. PET uses radionuclides known as positron emitters.
PET radiometals
Molecular imaging capabilities of PET
Beta particles
This type of radioactive decay involves the emission of a beta particle (electron) and is generally used for therapy since the beta particle only travels short distances in biological tissues. The resulting biologically damaging collisions between the particle and cellular components close to the radiopharmaceutical limiting damage to healthy surrounding tissues.
Beta particles from different sources contain a variety of ranges as follows:
- Low-range beta sources (mean range <200 µm). Nuclides of this category may provide a superior uniformity of deposit local energy over alpha emitters.
- The medium-range beta sources (200 µm < mean range < 0.2-1.0 mm) include the follow radionuclides: 47Sc, 67Cu, 77As, 105Rh, 109Pd, 111Ag, 161Tb and 186Re.
- This is in comparison to long-range beta sources (mean range > 1 mm) such as 32P, 90Y and 188Re.
Alpha particles
Alpha particles (helium nucleus) are used in therapy to destroy a variety of tumours and have the following associated properties: short-range in tissue (50-90 µm), irreparable DNA damage, potent single cell, cluster kill with high linear energy transfer (LET) of about 80 keV/µm. For example, an alpha particle transversing a diameter of a 10 µm cell nucleus will deposit energy of about 800 keV, which is equivalent to an absorbed dose of about 0.25 Gy.
However, 3-6 hits of alpha particles per cell nucleus are required to kill a fraction of 63% population. Potential alpha-emitters for radioimmunotherapy (RIT) are 211At and 212Bi: the latter being produced from 212Pb. Bismuth-212 has too short a half-life (60.6 min) to be used alone. Nevertheless, if a lead MoAb conjugation can be formed, 212Pb will continuously generate 212Bi acting on the tumour cells.
Electron capture
The process of Electron Capture (EC) and Internal Conversion (IC) decaying sources produce gamma rays and flux of Auger electrons. The majority of the emitted Auger electrons have a very short range (<1 µm). In this case, the source is attached or localised very close to the target DNA.
However, during the internal conversion (IC), the released electron is in a high energy state. EC or IC sources for radiolabelling of monoclonal antibodies with metal chelates – being the dominating bifunctional chelating agents – derivatives of DTPA, especially bicyclic anhydride of DTPA, have been widely used in labelling different proteins. These metal radionuclides include: 111In and to a lesser extent, 99mTc, 90Y, 67Ga, 68Ga, 109Pd, 46Sc, 186Re, 212Bi, and 169Yb.
Therapy radiometals
Criteria for the ideal radiometal
For a radiometal to be useful in medical applications, it must be in a stable coordination complex. This is usually achieved by binding the metal to a chelating ligand. To bind the metal effectively, the ligand needs specific properties. The most important are high thermodynamic stability and selectivity for the particular radionuclide. Other factors to be considered are the rate and method by which the complex is taken up and eliminated by the body, its ability to pass through membranes, and its toxicity. If the metal-ligand complex is not stable then the metal is released into the body.
For radiopharmaceutical use, the targeting agent has to be linked to the radiometal chelate complex. The chelate is described as bifunctional because of its ability to bind to the targeting moiety and also to complex the radiometal. The selection of the radiometal to tag onto an antibody via a macrocycle or BFC (bifunctional chelate) can act in two ways:
A bifunctional chelating agent possesses the following functionalities:
- The chelator acts like a ‘crab’ to bind the radiometal.
- The other part contains a chemically reactive functional group and binds to the N-terminal and ε-amines of lysines on the antibody.
- The radiometal if possible should be added last in the sequence before purification of the final product.
Radiometal chelate properties:
- The physical half-life of the radiometal must be between 6-200 hours (99mTc to 111In): It should be sufficiently long enough to allow for imaging at the time when the tumour to normal tissue ratio reaches a maximum. The time needed for the tumour to uptake the labelled monoclonal antibody is generally much longer 24-76 hours than that for normal organs to uptake common radiopharmaceuticals in nuclear medicine. If the half-life is too long, the radiometal creates an excess of unnecessary radiation dose to the patient.
- For imaging, the radiometal must have a gamma energy range of about 100-300 keV: The gamma-ray energy should match the scintigraph device (SPECT). In conventional nuclear medicine, a range of 100-300 keV is most appropriate for external scanning.
- High single energy gamma abundance per decay: A high photon density is desired for achieving high imaging resolution.
- No emission of particles, or low abundance of low-energy particle radiation. Any accompanying beta-particle will contribute to a considerable dose for the patient.
- The stable daughter product of the radiometal. The daughter nuclide will not only deliver an additional dose but also obscure the image.
- Production in carrier-free form (high specific activity). Since the number of binding sites of the protein or protein conjugating chelate molecule for the radionuclide is limited, only a carrier-free radionuclide can yield a labelled antibody of high specific activity, which is necessary to yield a clear image.
- Satisfactory in vivo chemical stability of the label or radiometal-protein complex. The in vivo chemical stability depends upon the following variables: bond energy of radiometal-protein or radiometal-chelate- protein, thermodynamic properties and pharmacokinetics
The metal is linked to the biomolecule via a bifunctional chelating agent (BFC). The BFC comprises two parts: it contains one or more functional groups that tightly complex the radiometal and an additional functional group that attaches to the biomolecule. Chelators most commonly used are the DTPA, DOTA & TETA derivatives.
- Radiometals require chelation chemistry or bifunctional chelators for linkage to antibodies
- Cyclic dianhydride derivatives
- 1B4M-DTPA (MX-DTPA, tiuxetan)
- CHX-A’DTPA: [effective chelator for 111In(γ), 90Y(β-), 177Lu(β-), 99mTc(γ)]
- DTPA derivatives can form a stable complex with bismuth radiometals conjugated to mAbs or peptides in vivo
- Resulting in radioimmunology conjugates (RIC’s) that have been used effectively in clinical trials
DOTA (indium, yttrium, lanthanides and actinides) and DTPA are suitable BFC for 3+ metals. DTPA is an effective chelating ligand for 90Y, 153Sm, 111In and 212Bi coordination through 3 amino nitrogens and five carboxylic oxygens atoms; hence, having a spare carboxylic acid group to link to the antibody. The best BFC for 177Lu is DOTA and its analogues.
A single carboxylic acid group is used to link to a biomolecule (peptide, antibody or drug): this includes the DOTA, DTPA and TETA derivatives. The remaining carboxylic acid groups in the DOTA, DTPA and TETA derivatives and the amine residues are used to bind the radiometal.
Antibodies
Radiolabelled antibodies have been used for detecting cancer since the early 1970s. This marked the beginning of the use of radiolabelled proteins and peptides for targeting receptors on tumours. Initially, antibodies were labelled with iodine radionuclides whereas today the use of radiometal-BFC-antibody conjugates are becoming more common, for example, Zevalin.
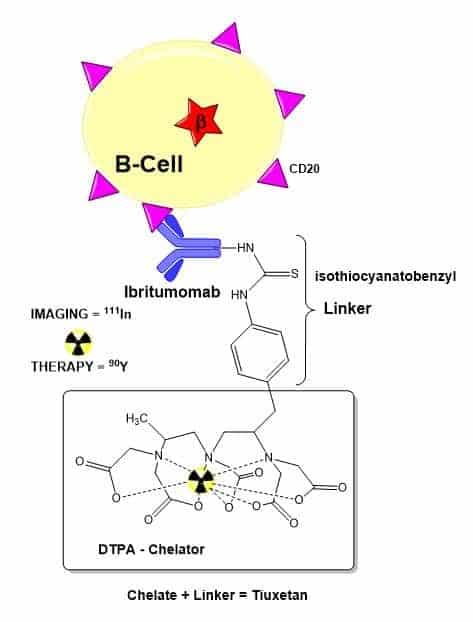
Zevalin – better together
Radiolabelled monoclonal antibody 90Y-ibritumomab tiuxetan (Zevalin):
- The Zevalin antibody is first labelled with the radiometal 111In – a gamma-emitter – and imaged using SPECT to verify that the antibody properly distributes within the body.
- In about 1% of cases, the distribution of the drug is altered (eg from excessive uptake in the bone marrow or kidney).
- If drug biodistribution is satisfactory, treatment is continued using a version of the Zevalin antibody labelled with the radiometal 90Y, a beta-emitter.
- The radioactive Yttrium can supply a lethal dose of radiation directly to the bound B-cells, and indirectly to neighbouring B-cells.
Peptides
- Peptide precision the hormone somatostatin is a peptide containing 14 amino acids. In a large number of human tumours, somatostatin receptors (SSRs) are found in larger numbers than they are in normal tissue. These are known as SSR-positive tumours. Targeting the SSRs with a radionuclide-labelled somatostatin molecule could therefore help image and treat tumours. Unfortunately, somatostatin has a very short biological half-life, so longer-lasting analogues have been developed to rectify this.
- Octreotide, which contains eight amino acids, is a cyclic analogue of somatostatin. When conjugated to DTPA and labelled with 111In, it forms the drug pentetreotide, the first peptide-based tumour imaging agent approved for human use in the US and Europe.
- Octreotide and other somatostatin analogues (such as RC-160 and several others developed by Diatide) have been labelled with various metal radionuclides via a number of different BFCs. The resulting complexes have been used both in imaging and therapy.
- For example, octreotide has been labelled with 67Ga and 68Ga using the BFC desferrioxamine-B (DFO): this is a well-known chelate ligand with a high affinity for Fe(III).
Imaging and therapy radiometal table
Conclusion
SPECT and PET imaging will continue to play a vital role in cancer theranostics; for example, monoclonal antibodies have been labelled with several radiometals such as 111In, 89Zr, 90Y and 177Lu. The overall aim is to provide diagnostic information with the therapeutic effect of the pharmaceutical to deliver the most effective approach towards the patients’ cancer treatment plan. Advancements in nuclear medicine allow for the ability to perform non-invasive methods to visualise drug target expression across primary and metastatic sites. Also, to evaluate the pharmacokinetics and efficacy of the therapeutic agents used. Currently, 68Ga has become a standard PET radiometal in the clinical setting because of the use of 68Ge/68Ga-generators. Investigations are continuing with the radiometals 64Cu and 89Zr to establish PET imaging applications especially in combination with theranostic approaches.
You are here: home » diagnostic medical imaging blog »