- How can you determine what’s in a closed box?
- How do CT scanners work?
- Hounsfield units (HU)
- Computed tomography scanner advancements
- CT systems
- 4-,8-, 32-, 40-, 64-, 128-, 256-, 320-, 640-CT Scanners
- Coverage area versus slices
- Mars scanner
- How much does a computed tomography scanner cost?
- The Future
Conventional X-ray systems use an immovable X-ray tube, whereas the computed tomography scanner uses a rotational X-ray source.
How can you determine what’s in a closed box?
The computed tomography scanner was invented in 1972 by Godfrey Hounsfield, a British engineer at EMI Laboratories in England, and the physicist Allan Cormack of Tufts University in Massachusetts.
This question was proposed in 1967 when the British electrical engineer Sir Godfrey Hounsfield (1919-2004) was walking in the countryside. Initially, this thought had nothing to do with medicine but was merely an idea that you could determine what was in a box by taking X-ray readings at all angles around the object. In order to develop the concept further, he assembled a computer to obtain position points by focusing X-rays at various angles to create an image of the hidden object.
Subsequently, the prototype head scanner emerged and was first tested on a preserved human brain, followed by a cow brain and Hounsfield himself. However, the first clinical computed tomography (CT) scan on a patient occurred on 1st October 1971 at Atkinson Morley’s Hospital (part of St George’s Hospital) in London, England. The patient had a suspected frontal lobe tumour and was scanned with the prototype scanner, which produced an image. This 80 x 80 matrix image was obtained by scanning the patient every 5 minutes and generated the image below:
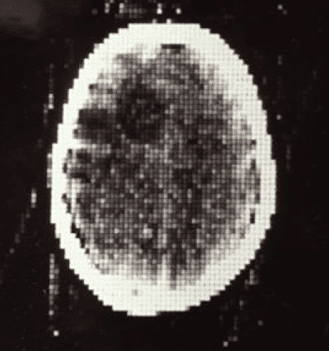
In 1975, Hounsfield built a whole-body scanner, which became available a year later. Using the most advanced multi-slice system, an entire chest scan can be taken in 5 to 10 seconds. These achievements were recognised by both pioneers, who won the 1979 Nobel Prize for Physiology and Medicine for developing the diagnostic techniques of X-ray computed tomography.
Computed tomography scanners have improved patient comfort since a scan can be performed quickly. Improvements have led to higher-resolution images, which assist the doctor in making a diagnosis. For example, the computed tomography scan can help doctors visualise small nodules or tumours, which they cannot see with a film X-ray.
It is a fact that in 2017 the NHS of England carried out 42.1 million imaging tests. These consisted of plain radiography (X-ray), of which there were 22.9 million procedures, diagnostic ultrasonography (ultrasound, 9.37 million); computerised axial tomography (CAT scan, 4.82 million) and magnetic resonance imaging (MRI, 3.36 million). In comparison, in 2018, it was estimated that approximately 82 million CT procedures were performed in the U.S.
How do CT scanners work?
Computed tomography scanners use X-rays (photons) that travel through the body, absorbing a certain amount of energy. The amount of X-ray energy absorbed is related to the slice thickness and proportional to the tissue density. Therefore, by changing the angle of the X-ray, the tissue densities can be correlated into a cross-sectional image using a computer. In these cases, the processed images are in a greyscale format. These shades of grey can correlate to the tissue density to create the image. The greyscale can be correlated to the Hounsfield scale, which allows a quantitative measure for describing radiodensity in the CT image and is able to calculate an accurate density for a particular type of tissue.
Hounsfield units (HU)
The CT attenuation values are expressed in Hounsfield units (HU) and are related to the linear density. In the Hounsfield scale, water is assigned a value of 0 HU, and all other CT values are formulated according to the following expression:
CT number (HU) = 1000 × (μmaterial – μwater) / (μwater)
μ is the CT linear attenuation coefficient
Therefore,
- HUwater = 0 as (µmaterial = µwater)
- HUair = -1000 as (µmaterial = 0)
- HU=1 is associated with 0.1% of the linear attenuation coefficient of water.
The HU values for each pixel represent the electron density of the imaged tissue at a particular location. These pixels are transformed into a digital image by assigning a greyscale intensity to each value. Therefore, higher HU values will produce a greater pixel intensity (brightness). For example, fat is less dense than water, and the associated HU value is in the −30 to −70 range thus, fat will appear darker than water in the computed tomography images. The table below shows several HU values for the various constituents which are found on head CT scans:
For example, on the Hounsfield scale:
- air is assigned a value of <−1000 (black on the greyscale)
- the bone between +700 (cancellous bone) to +3000 (dense bone) (white on the greyscale)
The bones are much denser than the surrounding soft tissues and appear very clearly on computed tomography images. Therefore, this makes computed tomography a vital imaging modality when investigating skeletal anatomy. Similarly, the density difference between soft tissues and the air is very good, allowing, for example, the nasal airways to be clearly seen. However, organs and soft tissues produce small Hounsfield value ranges, making it difficult to identify adjacent structures. For example, there is a difference between fat and muscle when viewing a segment of CT data. Artificial contrast agents that absorb X-ray energy may be introduced into the body, which makes some structures stand out more vividly in computed tomography images.
Conventional X-ray systems are based on an immovable X-ray tube, whereas the CT scanner uses a rotational X-ray source that revolves in an unclosed gantry. During the CT scanning process, the patient lies on a moveable bed, which transports through the gantry, allowing the narrow beams of X-rays to pass through the body at various angles during the rotation. Consequently, the X-ray detectors – located directly opposite the X-ray source – transmit to a computer to generate digital images.
Nevertheless, each time the X-ray source completes one full rotation, the CT computer constructs a 2-D image slice of the patient’s anatomy. The thickness of the tissue represents an image slice, which varies depending on the type of computed tomography machine used but usually ranges from 1-10 mm. Completing a full slice generates the image data; the motorised bed supporting the patient slowly moves forward into the gantry. The X-ray scanning process is repeated to produce another image slice. This process is repeated several times to accumulate enough slices. The benefit of this imaging process is the ability to rotate the 3D image in space. In addition, to be able to view slices in succession, which can make it simpler to locate the exact position of the abnormality within the body.
Currently, computed tomography scanners include technological developments that enable customers to improve patient care management. This includes lung cancer screening, dose guidance and regulation, spectral and multi-energy imaging, and cardiac and brain imaging. These CT scanners also provide new levels of information to help clinicians make a more confident diagnosis at a low dose without increasing complexity in their routines.
Optimising the dose including standardising protocols will contribute to improvements in healthcare systems and ensure operational efficiency and most importantly patient well-being.
The US computed tomography market has shown consistent growth over the last three years: this has been primarily driven by the Big Data and population health management systems.
Apart from dose reduction there is an important emphasis on workflow and efficiency to create improvements in the imaging experience for both patients and healthcare professionals.
Trends are being created in the area of preventative screening such as lung cancer.
The computed tomography scanner is capable of dual-energy and spectral imaging to obtain functional information from the same computed tomography scanner. The benefits of this approach are that patients will not be exposed to an additional radiation dose, and valuable functional information is obtained regarding the disease state: for example, the detection of lesions formed earlier in the disease process and also to assist in a personalised treatment plan. These concepts can be extended to structural heart imaging.
Computed tomography scanner advancements
The advancements in CT technology are focused on three areas:
- The capability to obtain vital clinical information from the CT investigation. The aim is to produce a more confident diagnosis, resulting in less follow-up testing and improved disease management.
- To develop methods to extract clinical information at lower radiation doses. This would enable broader use of CT for high-risk populations and early disease detection, such as lung cancer screening.
- The integration of clinical information across various diagnostic systems to benefit the individual patient treatment plan.
However, to enable clinical excellence for patients with complex disease states, computed tomography systems must be able to develop advanced modalities in spectral imaging technology for the diagnosis and characterisation of disease states. Furthermore, CT is mostly used to gain anatomical information and is expressed in tissue density (HU). This is compared to GE Healthcare – Gemstone Spectral Imaging (GSI), which can introduce additional contrast to the image to aid the diagnosis of complex disease patterns in the CT images. The GSI system overcomes the limitations of conventional CT, which adopts a single-parameter imaging mode. GSI will enable the acquisition of polychromatic images, optimal monochromatic images, iodine (water)-based images, and spectral characterisation diagrams. Therefore, GSI achieves high-resolution imaging and material decomposition by qualitative and quantitative analysis, significantly improving diagnostic accuracy and patient safety.
The Gemstone Spectral Imaging (GSI) process improves image quality compared to conventional imaging techniques. This is due to the higher contrast-to-noise ratio (CNR) and reduced beam hardening artefacts. In addition, enhanced material separation and quantitative material information are performed, all at a full 50 cm field of view.
GSI can provide vital information regarding the chemical composition of body materials. For example, distinguishing between calcium, iodine, and water helps in the characterisation of pathology. The GSI approach can be applied across several areas of clinical diagnosis, including:
- Enhancing contrast quality with the monochromatic spectral image
- Beam hardening reduced myocardial perfusion assessment
- Improved coronary visualisation in the presence of calcification
- Quantitative lesion characterisation
GSI is a dual-energy technique that uses fast kV switching, including gemstone detector technology, to generate material density data. It simultaneously acquires high and low-energy data sets to produce excellent anatomical information throughout the full 50 cm field of view. However, GSI decreases costs by reducing the requirement for other tests, primarily in the areas of cancer, vascular disease, and kidney stones.
By 2022, the computed tomography scanner market is estimated to be worth $6.5 billion. This has also been attributed to advancements in the area of 3D printing. In the future, the new CT scanners will incorporate the stereolithography file format, which will be used in 3D-printed structures as a standard for CT and MR applications of the technology. Therefore, 3D printers connected to CT scanners can print 3D models of human organs, such as the heart, to assist in preparing for complex surgery and training. CT scanning takes hundreds of X-ray images of the affected region as they are rotated inside the scanner. The 2D images are combined to produce a 3D point cloud, and the computed tomography image can then be compared to the CAD file to measure the accuracy of the print.
CT systems
The Siemens portfolio of CT scanners ranges from the 16-slice SOMATOM Scope up to the SOMATOM Force, which is capable of dual-energy imaging and is NEMA XR-29 compliant.
NEMA XR-29 specifies four attributes of CT scanners that optimise and manage radiation doses to deliver the diagnostic image quality the clinician needs.
The four criteria specified by NEMA XR-29 are:
- Dose check features
- Referencing adult and paediatric protocols
- Automatic exposure control
- DICOM-compliant radiation dose structured reporting
The equipment advancements continue with GE Healthcare by developing Hepatic VCAR, which enables whole organ segmentation within a minute compared to a standard CT scanner, which would be 10 minutes. This system will streamline the workflow and improve patients’ CT scanning output.
Furthermore, the TAVI (transcatheter aortic valve implantation) analysis assists physicians in treating sick patients with an intuitive, non-invasive planning tool for interventional surgical teams. Also, the Gemstone Clarity detector platform can have anatomical coverage in a single rotation, reducing the radiation dose required to obtain quality images.
4-,8-, 32-, 40-, 64-, 128-, 256-, 320-, 640-CT Scanners
The Toshiba Aquilion One Vision system is amongst the new generation of CT systems currently on the market. This scanner has the ability to offer several dose-lowering technologies, including advancements in hardware and software to enhance image quality over previous CT scanners. Consequently, healthcare institutions have begun to replace the first-generation 64-slice CT scanners with this consideration taken into account:
Do more slices make for a better CT scanner?
The answer is that the overall costs versus the benefits must be understood when purchasing high-slice CT systems. Accordingly, the CT scanner must be able to perform for that particular healthcare institution. For example, if it were to be used for cardiovascular imaging, the technical aspects of CT must have high image quality and resolution.
Coverage area versus slices
Among healthcare professionals, there is confusion about whether more slices on a CT scanner mean improved images. Evaluating a computed tomography scanner should involve questioning the detector area coverage – primarily measuring how much of the anatomy is being imaged simultaneously. It is essential that the greater imaging area covered determines the amount of stitching required for the images of a particular organ. The less stitching of images will lead to a reduction of artefacts that otherwise would require more time to reconstruct and review images. This is a problem in the movement of the heart and lungs.
The detector area coverage can vary between scanners with the same number of slices because the detectors on each machine are different in size.
For example, the 64-slice systems can range between 19.5 and 40 mm for detector area coverage. However, a system is considered a wide-area detector with an 8 cm coverage or greater. Wide detector systems tend to produce higher sensitivity associated with iterative reconstruction software. This computer software can improve both contrast and spatial resolutions through more powerful workstations.
Healthcare professionals think more slices are better because they do not have sound knowledge of physics or the technology involved in CT scanning. However, there is another consideration when carrying out high-end CT imaging. For example:
Mars scanner
Phil Butler and Anthony Butler invented the MARS spectral X-ray scanner at the Universities of Canterbury and Otago, New Zealand. It was used to scan a patient and to produce 3-D colour medical images. The MARS spectral X-ray scanner can potentially revolutionise medical imaging by diagnosing and treating many disease states, such as cancer and heart disease. This computed tomography system provides greater detail of the chemical components within the human body. A clinical trial using the machine to scan orthopaedic and rheumatology patients is planned. The technology used to develop the MARS CT scanner was based on the applications of CERN to locate the Higgs boson particle. The MARS CT scanner measures the X-ray spectrum to produce colour images instead of black-and-white ones. It is able to distinguish between various components within the body, such as fat, water, calcium and disease markers.
However, conventional black-and-white X-rays only allow measurement of the density and shape of an object.
A small version of the MARS scanner has been used to study cancer, bone and joint health, including vascular diseases that can cause heart attacks and strokes. The promising early results have indicated that spectral imaging will enable more accurate diagnosis and provide personalised treatment.
How much does a computed tomography scanner cost?
CT scanner prices vary considerably, including 16-slice, 64-slice, 128-slice, and 256-slice models. The price ranges are based on major manufacturers’ new and refurbished CT scanners.
The Future
The next generation of computed tomography scanners will be faster, fully automated and easier to use. This technology platform will depend on transitioning from standard CT machines to more advanced systems, improving quantification and quicker diagnosis. The overall emphasis is to assist medical imaging professionals in limiting the patient’s length of stay, thereby reducing cost and improving efficiency. These future CT scanners will be able to consistently deliver anatomical information and contain the ability to characterise anatomical structures within a single scan. Hence, future systems will contribute to clinical pathways providing individual personalised treatment plans. Nevertheless, these future CT advancements require Big Data, and therefore, cloud computing will be used to process the considerable amount of data generated by a single CT scan.
Currently, the GE Health Cloud has enormous potential to analyse data from a CT scan, enabling clinicians to formulate a more accurate response to the treatment of the disease state.
The future of CT scanning will be about how to manage massive data sets during image processing.
Another CT growth area will be in chest pain management. In these cases, it is paramount for the patient to undergo evaluation quickly to reduce the time spent in the emergency department and to facilitate a safe discharge. The healthcare sector currently produces vast amounts of diagnostic imaging data but does not have the resources to process it all. Future healthcare professionals must be able to understand CT technology fully. Also, they must be confident in quantifying and making sense of the generated data. Furthermore, and most importantly, the goal is to transfer the results to the patient meaningfully, producing more comprehensive treatment plans and reducing overall costs.
You are here: home » medical imaging blog »